Epidemiology, pathophysiology, and microbiology of community-acquired pneumonia
Introduction
Community-acquired pneumonia (CAP) remains the main cause of death from infectious disease globally and is associated with considerable impact on morbidity and mortality especially in the elderly. Several studies have also indicated that its incidence has risen over recent decades, and that more patients now require hospitalization. However, there is no clear explanation for this phenomenon (1-4). Important contributors to the growing incidence are increased life expectancy, multiple chronic diseases, and immunosuppression. The incidence and risk of death associated with CAP are linked to increasing age. Indeed, older age is an important risk factor for pneumonia and is associated with elevated morbidity and mortality, due not only to the physiological changes associated with aging but also the higher rate of chronic disease (5). Although it is true that CAP-related mortality has declined over time in the general population (6), rates remain as high as 35% in patients with severe CAP requiring intensive care unit (ICU) admission (7).
Globally, Streptococcus pneumoniae (pneumococcus) is the most frequent causative pathogen in CAP, regardless of setting (i.e., outpatients, inpatients, and ICU admissions), age group, and comorbidity (8,9). However, improvements in molecular diagnostic techniques over recent years has shown that there is an increasing prevalence of respiratory viruses in CAP, especially in the United States (10,11). Also, the epidemiological changes that occurred because of serotype replacement after the introduction of pneumococcal conjugate vaccine is an emerging challenge, with some reports of multidrug-resistant (MDR) pneumococcal serotypes becoming problematic (12,13).
This review provides an overview of the main published data regarding CAP in the adult population.
Global epidemiology of CAP
The Global Burden of Disease study [2015] reported that lower respiratory infections are the third most common cause of death globally, exceeded only by ischemic heart disease and cerebrovascular disease (14). Similarly, statistics of World Health Organization in 2015 indicated that 3.2 million of the 56.4 million deaths worldwide in 2015 were caused by lower respiratory infections, making them the most deadly communicable disease and third-leading cause of mortality. Figure 1 summarizes the relevant data from the Global Burden of Disease study.
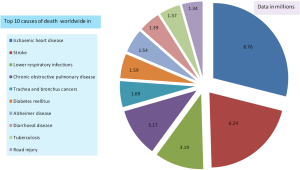
In the United States, community data reported by Jain et al. in 2015 (10) indicated that there was an increased incidence of pneumonia with increasing age. The authors estimated CAP had an incidence of 24.8 episodes per 10,000 adults, with the highest incidence in those aged 65–79 years (63 cases per 10,000 adults) and ≥80 years (164.3 cases per 10,000 adults). In a recently published study by Ramirez et al. (15), it was estimated that more than 1.5 million of adult patients are hospitalized each year in United States with CAP, and that 10,000 of these patients will die during hospitalization. This study also showed that approximately one in three adults will die within one year of being hospitalized with pneumonia.
There is a varied picture elsewhere in the world. In Europe, for example, the annual incidence of CAP has been estimated at 1.07–1.2 cases per 1,000 persons per year. This incidence increases with age to 14 cases per 1,000 person per year in patients aged ≥65 years. Also, the incidence of CAP was higher in men than in women (16). In an Asian multicenter surveillance study (17) the annual incidence for adult community-onset pneumonia (including CAP and healthcare-associated pneumonia) was estimated at 16.9 cases per 1,000 persons per year, again being higher in men than in women for all age groups (15.6 vs. 9.3 per 1,000 persons per year). Also, in a prospective hospital-based surveillance study by Takaki et al. (18), the incidence rates for CAP were 3.4, 10.7, and 42.9 per 1,000 persons per year in the 15–64 years age group, 65–74 years age group, and ≥75 years age group, respectively. However, there are only limited data for the incidence of CAP in Latin America, making it difficult to know the real impact of this disease. Only one report has looked at the clinical and economic burdens of pneumonia in Latin American adults, and indicated that the annual incidence rates in Argentina, Chile, and Brazil in 2010 were 120,000, 170,000, and 920,000 cases, respectively. According to a statistical health report on mortality in South Africa, influenza and pneumonia together were ranked the sixth leading cause of death in 2015 (19).
Pathophysiology: routes of microorganism spread into lung tissue
The infectious process resulting from the invasion and overgrowth of pathogenic microorganism in the lung parenchyma, combined with the breaking down of local defense mechanisms and the production of intra-alveolar exudates, gives a basic definition of pneumonia (20). There is a balance between pathogen-related factors (e.g., virulence and inoculum size) and host-related factors (e.g., sex, age, and comorbidities) in the development and severity of pneumonia.
Inhalation
Inhalation after exposure to microorganisms that survive suspended in air droplets before transport from the initial source to a susceptible host is an important and frequent mode of spread. It is reported that particles smaller than 5 µm can transport up to 100 microorganisms, depending on bacterial size, and thereby reach the alveoli by evading the respiratory host defenses. This is the most common route of infection in community infections among healthy young patients, and the usual route of spread of respiratory viruses and intracellular pathogens. The influenza virus is a good example of this route, starting with aerosol (droplet nuclei) transmission via water- and virus-laden respiratory droplets that are then exhaled by an infected person in a desiccated form allowing it to remain light enough to be suspended in the air for minutes to hours. These infectious aerosols can then be inhaled into the respiratory tract of a susceptible person and initiate infection. In the case of droplet spray transmission, an infected person coughs or sneezes, expelling respiratory droplets that contain contagious virus particles, which directly impact on the nasal mucosa of a susceptible person (21). Another example of bacterial transmission occurs with Legionella, which is usually transmitted by the inhalation of contaminated aerosols produced by water systems (e.g., cooling towers, showers, hot water distribution systems, and faucets).
Aspiration
Microparticles (≤5 µm) and microorganisms present in the upper airways are constantly exposed to lung tissue, and through micro-aspiration of oropharyngeal secretions from the trachea, can enter the lower airways. However, the lower airways retain defense mechanisms to avoid invasion of the alveoli in the lungs, so an innate or acquired defect is required to initiate pneumonia. The most important predisposing factors for aspiration are a depressed cough reflex, altered consciousness, an impaired mucociliary escalator system, and immunosuppression (22).
Nasopharyngeal carriage rates of S. pneumoniae in healthy children and adults range from 20% to 50% and from 5% to 30%, respectively (23,24). It is known that previous respiratory viral infection provides a vector for pneumococcus from the oropharynx to the lower respiratory tract, because viruses induce changes in the host respiratory tract (e.g., epithelial damage, changes in airway function, up-regulation and exposure of receptors) and innate immunity (25). Moreover, the eradication of serotypes included in conjugate vaccines in healthy carriers has created an ecological niche for new serotypes not included in the vaccines (serotype replacement).
In approximately 35% to 75% of hospitalized patients, their oropharynx is colonized with gram-negative microorganisms between 3 and 5 days of hospital admission, depending on the severity and type of previous underlying disease. The risk factors associated with airway colonization by these pathogens include previous antibiotic therapy, length of hospitalization, intubation, current smoker, heavy alcohol consumption, malnutrition, and dental plaque (22).
In a German study of bacterial colonization patterns in mechanically ventilated patients, the initial colonization rate at any site (e.g., nasal and pharyngeal, tracheobronchial, gastric, and protected-specimen brush sample) was 83% among brain-injured patients admitted to ICU. S. pneumoniae, Haemophilus influenzae, and Staphylococcus aureus were the predominant upper airway pathogens (26).
Hematogenous spread
Hematogenous spread is infrequent in pneumonia, but when present, occurs from an adjacent infection site, such as right-sided endocarditis or bacterial translocation from the stomach, to the blood, and then the lungs (27).
Direct extension from adjacent infected foci
Tuberculosis can spread contiguously from the lymph nodes to the pericardium or the lung, but this is only rarely a route of pneumonia formation.
Microbiology of CAP
In recent years, several studies have assessed the microbial etiology of CAP (8,10). Some indicate that the etiology of CAP differs by the severity of pneumonia at clinical presentation (8,28,29) (Table 1), while others indicate that there is a seasonal distribution (30) (Figure 2).
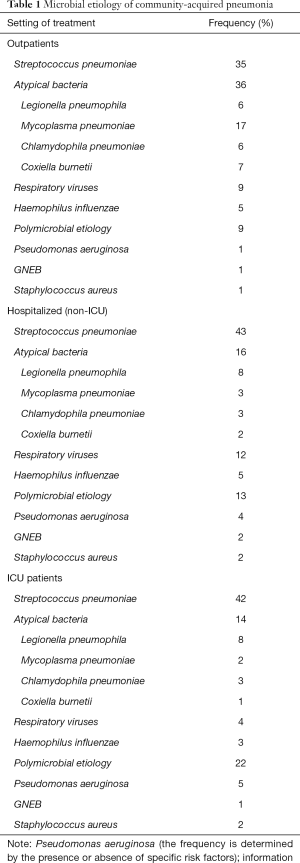
Full table
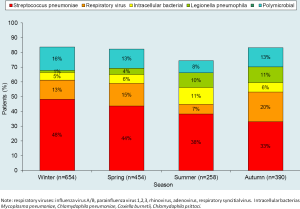
Microbiology of bacterial CAP
The pneumococcus remains the most frequent pathogen in CAP. However, its incidence has been decreasing in the United Stated and Canada due to the introduction of polysaccharide vaccines (e.g., PCV7 and PCV13) (31) and the decreased rate of smoking (32,33). By contrast, this decrease has not be observed in Europe (8,34-36).
An interesting study from Canada was published recently, looking at the impact of the pneumococcal vaccine on hospitalization due to pneumonia between 1992 and 2014 by the introduction of the pneumococcal vaccination. The included periods were as follows: pre-vaccine; PCV7 available for private purchase; public funding for the PCV7; replacement of the PCV7 with the PCV10; and replacement of the PCV10 with the PCV13. This revealed that of 1,063,700 hospitalizations for pneumonia during the study period, hospitalization for pneumonia declined by 34%, 38%, and 45% after public funding for PCV7, PCV10, and PCV13, respectively (33). However, an important issue regarding the pneumococcus and the introduction of pneumococcal conjugate vaccines is the phenomenon of pneumococcal serotype replacement. This occurs when the overall carriage of vaccine-covered serotypes decreases, and previously rare serotypes become more prevalent in the nasopharynx.
The phenomenon of pneumococcal serotype replacement has also changed the epidemiology of pneumococcal disease worldwide, with increasing resistance to several antibiotics (e.g., cephalosporins, macrolides and fluoroquinolones) observed globally over the last two decades (37,38). Mortality rates related to antibiotic-resistant S. pneumoniae in CAP have not increased since the introduction of the pneumococcal conjugate vaccine, which covers the serotypes most likely to express resistance (39). Also, the clinical relevance of macrolide resistance is unclear for the pneumococcus. In a prospective observational study, Cillóniz et al. compared clinical outcomes in hospitalized CAP patients with and without macrolide-resistant pneumococcus and reported no evidence of worse presentations or worse clinical outcomes among patients hospitalized with macrolide-resistant S. pneumoniae CAP if they were treated with guideline-compliant versus noncompliant regimens. The risk factors associated with antibiotic resistance in S. pneumoniae are summarized in Table 2.
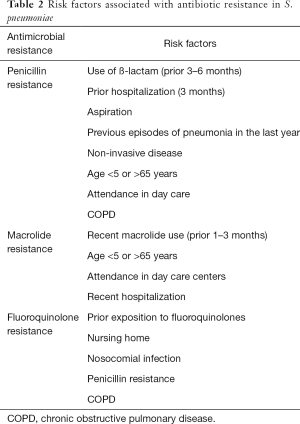
Full table
Macrolide resistance in S. pneumoniae ranges from 20% to 40%. Two mechanisms of macrolide resistance have been reported for pneumococcal pneumonia. In North America, the most frequent mechanism involves the efflux of the drug from the bacteria, conferring a low level of macrolide resistance. In Europe, the most frequent mechanism involves alterations at the level of the ribosomal target of antimicrobial action, which confers a high level of macrolide resistance. This is an all-or-nothing resistance mechanism: if patients are treated with macrolides, they will present with treatment failure.
The reported rate of fluoroquinolone resistance is higher in Europe (5.2%) than in the United States (1.2%) and Asia (2.4%) (40-43). Target site mutations—in topoisomerase genes (e.g., topoisomerase IV subunit parC), which are the principal targets for ciprofloxacin and levofloxacin, and in DNA gyrase (e.g., gyrA2 and gyrB2), which are the principal target for moxifloxacin—are the main mechanism of fluoroquinolone resistance to the pneumococcus. Fluoroquinolone resistance is rare in pediatric populations, but is higher in adults, especially in those older than 64 years and in those with chronic obstructive pulmonary disease (44). The risk factors for fluoroquinolone resistance in adults with pneumococcal pneumonia have been addressed by Kang et al. (45). In their research, it was shown that previous therapy with fluoroquinolones, cerebrovascular disease, and healthcare-associated infection were significantly associated with levofloxacin-nonsusceptible pneumococcal pneumonia (all P<0.05).
In the last decade, MDR pneumococcus, with resistance to at least three different classes of antibiotics, has rapidly emerged worldwide for some non-vaccine serotypes. Among the MDR serotypes, serotype 19A accounts for a high proportion, though a sustained decline in the incidence of serotype 19A was observed in all age groups after the introduction of PCV13 vaccine in several countries (46-48). However, new non-vaccine MDR serotypes have emerged, including serotypes 6B, 6C, 14, 15B/C, 19F, and 23A (49,50).
In CAP among outpatients and hospitalized patients, intracellular pathogens (e.g., Mycoplasma pneumoniae, Legionella pneumoniae, Chlamydophila pneumonia, Chlamydophila psittaci, and Coxiella burnetii) and pneumococcus are common causes (8,51,52). A large observational study of the microbial etiology of CAP in outpatients revealed that intracellular pathogens were the second most frequent cause of pneumonia (33% of patients with defined etiology) (28). In some cases, intracellular pathogens may cause more severe disease, presenting with respiratory failure and multisystem dysfunction, leading to fatal outcomes. In approximately 1% to 7% of cases, these microorganisms may cause severe CAP (51,53-55).
MDR pathogens are potentially a globally concern. Approximately 6% of CAP cases caused by MDR pathogens, S. aureus and P. aeruginosa being the frequently isolated (13,56). In two independent European cohorts hospitalized with CAP, it was shown that MDR pathogens were causative in 3.3% (a Spanish cohort) and 7.6% (an Italian cohort), with methicillin-resistant S. aureus (MRSA) being most common (13). CAP caused by MRSA has also been shown to be more frequent in areas with a high prevalence of community acquired MRSA infections (57).
In a prospective observational study of 2,023 adults in 2016 (58), Cillóniz et al. reported that CAP was due to P. aeruginosa in 77 of 2,023 cases (4%). MDR and non-MDR P. aeruginosa were presented in 32% and 68%, respectively. Risk factors for P. aeruginosa pneumonia in that study were male sex, chronic respiratory disease, C-reactive protein <12.35 mg/dL, and PSI risk class IV–V. By contrast, the only risk factor for MDR P. aeruginosa pneumonia was previous antibiotic therapy. The authors concluded that CAP due to P. aeruginosa was an independent risk factor for 30-day mortality.
There are several scoring systems for predicting MDR pathogens in CAP (Figure 3). In 2012, Aliberti et al. (59) proposed a score that included: chronic renal failure (5 points), prior hospitalization (4 points), nursing home residence (3 points), and other variables worth 0.5 points each (i.e., cerebrovascular disease, diabetes, chronic obstructive pulmonary disease, immunosuppression, home wound care, prior antimicrobial therapy, and home infusion therapy). The prevalence of resistant pathogens was 38% in patients with a score ≥3 points, compared with 8% in patients with a score of ≤0.5.
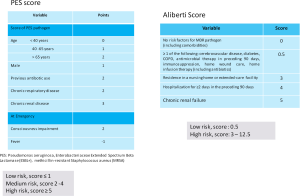
In 2015, Prina et al. (56) proposed a PES score based on the three most frequent MDR pathogens in CAP (e.g., P. aeruginosa, Enterobacteriaceae extended spectrum b-lactamase-positive, and methicillin-resistant S. aureus). This score was scored as follows: 1 point each for age 40–65 years and male sex; 2 points each for age >65 years, previous antibiotic use, chronic respiratory disorder, and impaired consciousness; 3 points for chronic renal failure; and minus 1 point if fever is present initially. Patients with 5 points or higher on the PES score were considered to be at increased risk of MDR pathogens.
Respiratory viruses and CAP
Respiratory viruses account for 7–36% of cases of CAP when the microbial etiology is defined (10,60,61). However, this incidence varies with seasonality, geographic location, and age. In a recent study by Jain et al. (10) on CAP in hospitalized adult patients from United States, the three principal pathogens related to CAP were rhinovirus (9%), influenza virus (6%), and S. pneumoniae (5%).
In recent studies, respiratory viruses have been reported with higher frequencies in cases with severe pneumonia (62) or complications like acute respiratory distress syndrome (63-66). It is known that seasonal bouts of the influenza virus and respiratory syncytial virus cause pneumonia in very young and very old patients (67,68), but it is now apparent that pandemic viruses mostly affect children and younger adults (10,69,70). Also, newer emerging respiratory viruses have been documented in the last decade, including human metapneumovirus (HMPV) in 2001 (71) and coronavirus in 2002 (72), with the latter responsible for severe acute respiratory syndrome. Other examples of recently discovered viruses include human bocavirus discovered in 2005 (73) and Middle East respiratory syndrome described in 2012 (74).
A recent systematic review and meta-analysis covered the respiratory viral pathogens among European adults with CAP between 2001 and 2015 (75). The study reported that viruses were mainly detected in at least 22% of patients hospitalized with radiologically confirmed CAP. However, this increased after 2010 when molecular diagnostic testing was incorporated and the proportion of respiratory viruses detected reached 25%. Influenza viruses, rhinoviruses, and coronaviruses were the most frequently identified respiratory viruses, accounting for over half of all cases.
Microbiome and CAP
Concepts about the sterility of lung tissue have changed since the introduction of new culture-independent methods has demonstrated that the lungs contain diverse microbial communities (76). These communities exist without causing infection, but there is a shift in their composition during disease (77). Several factors affect the composition of these communities, including immigration and elimination of microorganisms. When the microbiome becomes unbalanced in this way, dysbiosis is said to have occurred. An interesting example is how the microbiome is affected by antibiotics. In the study by Abeles et al. on the effects of short-term courses of amoxicillin and azithromycin on the human microbiota (78), the authors proposed “if antibiotics are often absorbed across the gastrointestinal tract and distributed to the tissues via the bloodstream, they would affect the microflora of each body surface tested (gut, skin and, mouth).” As few as 3 days’ antibiotic treatment caused sustained reductions in microbiota diversity, which may have implications for the maintenance of human health and resilience to disease.
A study of the microbiome in 45 sputum samples in patients with CAP reported similarities with healthy controls at the phylum level, with stability for Streptococcus spp. and Neisseria spp. at the genus level; however, Moraxella spp. and Rothia spp. had changed. The authors concluded that Rothia spp. may be an endogenous pneumonia-causing pathogen (79). Similarly, in a study of elderly patients with pneumonia, three microbiota profiles (lactobacilli, S. pneumoniae, and Rothia spp.) were strongly associated with pneumonia, suggesting that pneumonia in elderly patients is associated with dysbiosis of the upper respiratory tract.
Conclusions
CAP remains a significant cause of morbidity, mortality, and health costs globally, despite improvements in its management over the last decade. There has been a significant increase in the incidence of CAP in Europe over the past decade, probably because of the increasing age of the population and the greater number of comorbidities. It is hoped that advances in molecular diagnostic tests will help us detect the causative pathogens in pneumonia, especially antibiotic-resistant pathogens. Preventive strategies for CAP, including the surveillance of trends in microbial etiology, may help improve vaccination policies in this specific population.
Acknowledgements
None.
Footnote
Conflicts of Interest: The authors have no conflicts of interest to declare.
References
- File TM, Marrie TJ. Burden of community-acquired pneumonia in North American adults. Postgrad Med 2010;122:130-41. [Crossref] [PubMed]
- Trotter CL, Stuart JM, George R, et al. Increasing hospital admissions for pneumonia, England. Emerg Infect Dis 2008;14:727-33. [Crossref] [PubMed]
- Ewig S, Birkner N, Strauss R, et al. New perspectives on community-acquired pneumonia in 388 406 patients. Results from a nationwide mandatory performance measurement programme in healthcare quality. Thorax 2009;64:1062-9. [Crossref] [PubMed]
- Thomsen RW, Riis A, Nørgaard M, et al. Rising incidence and persistently high mortality of hospitalized pneumonia: a 10-year population-based study in Denmark. J Intern Med 2006;259:410-7. [Crossref] [PubMed]
- Cilloniz C, Ceccato A, San Jose A, et al. Clinical Management of Community Acquired Pneumonia in the Elderly Patient. Expert Rev Respir Med 2016. [Epub ahead of print]. [Crossref] [PubMed]
- Simonetti AF, Garcia-Vidal C, Viasus D, et al. Declining mortality among hospitalized patients with community-acquired pneumonia. Clin Microbiol Infect 2016;22:567.e1-7. [Crossref] [PubMed]
- Blasi F, Mantero M, Santus P, et al. Understanding the burden of pneumococcal disease in adults. Clin Microbiol Infect 2012;18 Suppl 5:7-14. [Crossref] [PubMed]
- Cillóniz C, Ewig S, Polverino E, et al. Microbial aetiology of community-acquired pneumonia and its relation to severity. Thorax 2011;66:340-6. [Crossref] [PubMed]
- Cillóniz C, Polverino E, Ewig S, et al. Impact of age and comorbidity on cause and outcome in community-acquired pneumonia. Chest 2013;144:999-1007. [Crossref] [PubMed]
- Jain S, Self WH, Wunderink RG, et al. Community-Acquired Pneumonia Requiring Hospitalization. N Engl J Med 2015;373:2382. [Crossref] [PubMed]
- Burk M, El-Kersh K, Saad M, et al. Viral infection in community-acquired pneumonia: a systematic review and meta-analysis. Eur Respir Rev 2016;25:178-88. [Crossref] [PubMed]
- Cillóniz C, Ardanuy C, Vila J, et al. What is the clinical relevance of drug-resistant pneumococcus? Curr Opin Pulm Med 2016;22:227-34. [Crossref] [PubMed]
- Aliberti S, Cilloniz C, Chalmers JD, et al. Multidrug-resistant pathogens in hospitalised patients coming from the community with pneumonia: a European perspective. Thorax 2013;68:997-9. [Crossref] [PubMed]
- GBD 2015 Mortality and Causes of Death Collaborators. Global, regional, and national life expectancy, all-cause mortality, and cause-specific mortality for 249 causes of death, 1980-2015: a systematic analysis for the Global Burden of Disease Study 2015. Lancet 2016;388:1459-544. [Crossref] [PubMed]
- Ramirez JA, Wiemken TL, Peyrani P, et al. Adults Hospitalized with Pneumonia in the United States: Incidence, Epidemiology & Mortality. Clin Infect Dis 2017;65:1806-12. [PubMed]
- Torres A, Peetermans WE, Viegi G, et al. Risk factors for community-acquired pneumonia in adults in Europe: a literature review. Thorax 2013;68:1057-65. [Crossref] [PubMed]
- Morimoto K, Suzuki M, Ishifuji T, et al. The burden and etiology of community-onset pneumonia in the aging Japanese population: a multicenter prospective study. PloS One 2015;10:e0122247. [Crossref] [PubMed]
- Takaki M, Nakama T, Ishida M, et al. High incidence of community-acquired pneumonia among rapidly aging population in Japan: a prospective hospital-based surveillance. Jpn J Infect Dis 2014;67:269-75. [Crossref] [PubMed]
- Boyles TH, Brink A, Calligaro GL, et al. South African guideline for the management of community-acquired pneumonia in adults. J Thorac Dis 2017;9:1469-502. [Crossref] [PubMed]
- Alcón A, Fàbregas N, Torres A. Pathophysiology of pneumonia. Clin Chest Med 2005;26:39-46. [Crossref] [PubMed]
- Thangavel RR, Bouvier NM. Animal models for influenza virus pathogenesis, transmission, and immunology. J Immunol Methods 2014;410:60-79. [Crossref] [PubMed]
- Nair GB, Niederman MS. Nosocomial pneumonia: lessons learned. Crit Care Clin 2013;29:521-46. [Crossref] [PubMed]
- Bogaert D, De Groot R, Hermans PW. Streptococcus pneumoniae colonisation: the key to pneumococcal disease. Lancet Infect Dis 2004;4:144-54. [Crossref] [PubMed]
- Ferreira DM, Jambo KC, Gordon SB. Experimental human pneumococcal carriage models for vaccine research. Trends Microbiol 2011;19:464-70. [Crossref] [PubMed]
- McCullers JA. Insights into the interaction between influenza virus and pneumococcus. Clin Microbiol Rev 2006;19:571-82. [Crossref] [PubMed]
- Ewig S, Torres A, El-Ebiary M, et al. Bacterial colonization patterns in mechanically ventilated patients with traumatic and medical head injury. Incidence, risk factors, and association with ventilator-associated pneumonia. Am J Respir Crit Care Med 1999;159:188-98. [Crossref] [PubMed]
- Rumbak MJ. The pathogenesis of ventilator-associated pneumonia. Semin Respir Crit Care Med 2002;23:427-34. [Crossref] [PubMed]
- Cillóniz C, Ewig S, Polverino E, et al. Community-acquired pneumonia in outpatients: aetiology and outcomes. Eur Respir J 2012;40:931-8. [Crossref] [PubMed]
- Marrie TJ, Poulin-Costello M, Beecroft MD, et al. Etiology of community-acquired pneumonia treated in an ambulatory setting. Respir Med 2005;99:60-5. [Crossref] [PubMed]
- Cilloniz C, Ewig S, Gabarrus A, et al. Seasonality of pathogens causing community-acquired pneumonia. Respirology 2017;22:778-85. [Crossref] [PubMed]
- Moberley S, Holden J, Tatham DP, et al. Vaccines for preventing pneumococcal infection in adults. Cochrane Database Syst Rev 2013.CD000422. [PubMed]
- Centers for Disease Control and Prevention (CDC). Current cigarette smoking among adults - United States, 2011. MMWR Morb Mortal Wkly Rep 2012;61:889-94. [PubMed]
- Luca DL, Kwong JC, Chu A, et al. Impact of Pneumococcal Vaccination on Pneumonia Hospitalizations and Related Costs in Ontario: A Population-Based Ecological Study. Clin Infect Dis 2017. [Epub ahead of print]. [Crossref] [PubMed]
- Johansson N, Kalin M, Tiveljung-Lindell A, et al. Etiology of community-acquired pneumonia: increased microbiological yield with new diagnostic methods. Clin Infect Dis 2010;50:202-9. [Crossref] [PubMed]
- Rozenbaum MH, Pechlivanoglou P, van der Werf TS, et al. The role of Streptococcus pneumoniae in community-acquired pneumonia among adults in Europe: a meta-analysis. Eur J Clin Microbiol Infect Dis 2013;32:305-16. [Crossref] [PubMed]
- Huijts SM, Pride MW, Vos JM, et al. Diagnostic accuracy of a serotype-specific antigen test in community-acquired pneumonia. Eur Respir J 2013;42:1283-90. [Crossref] [PubMed]
- Cilloniz C, Albert RK, Liapikou A, et al. The Effect of Macrolide Resistance on the Presentation and Outcome of Patients Hospitalized for Streptococcus pneumoniae Pneumonia. Am J Respir Crit Care Med 2015;191:1265-72. [Crossref] [PubMed]
- Van Bambeke F, Reinert RR, Appelbaum PC, et al. Multidrug-resistant Streptococcus pneumoniae infections: current and future therapeutic options. Drugs 2007;67:2355-82. [Crossref] [PubMed]
- Stephens DS, Zughaier SM, Whitney CG, et al. Incidence of macrolide resistance in Streptococcus pneumoniae after introduction of the pneumococcal conjugate vaccine: population-based assessment. Lancet 2005;365:855-63. [Crossref] [PubMed]
- Jones RN, Sader HS, Mendes RE, et al. Update on antimicrobial susceptibility trends among Streptococcus pneumoniae in the United States: report of ceftaroline activity from the SENTRY Antimicrobial Surveillance Program (1998-2011). Diagn Microbiol Infect Dis 2013;75:107-9. [Crossref] [PubMed]
- Kim SH, Song JH, Chung DR, et al. Changing trends in antimicrobial resistance and serotypes of Streptococcus pneumoniae isolates in Asian countries: an Asian Network for Surveillance of Resistant Pathogens (ANSORP) study. Antimicrob Agents Chemother 2012;56:1418-26. [Crossref] [PubMed]
- Chen HH, Li HC, Su LH, et al. Fluoroquinolone-nonsusceptible Streptococcus pneumoniae isolates from a medical center in the pneumococcal conjugate vaccine era. J Microbiol Immunol Infect 2017;50:839-45. [Crossref] [PubMed]
- Ceyssens PJ, Van Bambeke F, Mattheus W, et al. Molecular Analysis of Rising Fluoroquinolone Resistance in Belgian Non-Invasive Streptococcus pneumoniae Isolates (1995-2014). PLoS One 2016;11:e0154816. [Crossref] [PubMed]
- de la Campa AG, Ardanuy C, Balsalobre L, et al. Changes in fluoroquinolone-resistant Streptococcus pneumoniae after 7-valent conjugate vaccination, Spain. Emerg Infect Dis 2009;15:905-11. [Crossref] [PubMed]
- Kang CI, Song JH, Kim SH, et al. Risk factors for levofloxacin-nonsusceptible Streptococcus pneumoniae in community-acquired pneumococcal pneumonia: a nested case-control study. Eur J Clin Microbiol Infect Dis 2014;33:55-9. [Crossref] [PubMed]
- Moore MR, Link-Gelles R, Schaffner W, et al. Effect of use of 13-valent pneumococcal conjugate vaccine in children on invasive pneumococcal disease in children and adults in the USA: analysis of multisite, population-based surveillance. Lancet Infect Dis 2015;15:301-9. [Crossref] [PubMed]
- Collins DA, Hoskins A, Snelling T, et al. Predictors of pneumococcal carriage and the effect of the 13-valent pneumococcal conjugate vaccination in the Western Australian Aboriginal population. Pneumonia (Nathan) 2017;9:14. [Crossref] [PubMed]
- Regev-Yochay G, Katzir M, Strahilevitz J, et al. The herd effects of infant PCV7/PCV13 sequential implementation on adult invasive pneumococcal disease, six years post implementation; a nationwide study in Israel. Vaccine 2017;35:2449-56. [Crossref] [PubMed]
- Lee JK, Yun KW, Choi EH, et al. Changes in the Serotype Distribution among Antibiotic Resistant Carriage Streptococcus pneumoniae Isolates in Children after the Introduction of the Extended-Valency Pneumococcal Conjugate Vaccine. J Korean Med Sci 2017;32:1431-9. [Crossref] [PubMed]
- Golden AR, Rosenthal M, Fultz B, et al. Characterization of MDR and XDR Streptococcus pneumoniae in Canada, 2007-13. J Antimicrob Chemother 2015;70:2199-202. [Crossref] [PubMed]
- Cillóniz C, Torres A, Niederman M, et al. Community-acquired pneumonia related to intracellular pathogens. Intensive Care Med 2016;42:1374-86. [Crossref] [PubMed]
- Lui G, Ip M, Lee N, et al. Role of “atypical pathogens” among adult hospitalized patients with community-acquired pneumonia. Respirology 2009;14:1098-105. [Crossref] [PubMed]
- Capelastegui A, España PP, Bilbao A, et al. Etiology of community-acquired pneumonia in a population-based study: link between etiology and patients characteristics, process-of-care, clinical evolution and outcomes. BMC Infect Dis 2012;12:134. [Crossref] [PubMed]
- Miyashita N, Obase Y, Ouchi K, et al. Clinical features of severe Mycoplasma pneumoniae pneumonia in adults admitted to an intensive care unit. J Med Microbiol 2007;56:1625-9. [Crossref] [PubMed]
- Woodhead M. Community-acquired pneumonia in Europe: causative pathogens and resistance patterns. Eur Respir J Suppl 2002;36:20s-27s. [Crossref] [PubMed]
- Prina E, Ranzani OT, Polverino E, et al. Risk Factors Associated with Potentially Antibiotic-Resistant Pathogens in Community-Acquired Pneumonia. Ann Am Thorac Soc 2015;12:153-60. [Crossref] [PubMed]
- Obed M, García-Vidal C, Pessacq P, et al. Clinical features and outcome of community-acquired methicillin-resistant Staphylococcus aureus pneumonia. Enferm Infecc Microbiol Clin 2014;32:23-7. [Crossref] [PubMed]
- Cillóniz C, Gabarrús A, Ferrer M, P, et al. Community-Acquired Pneumonia due to Multidrug and non-Multidrug resistant Pseudomonas aeruginosa. Chest 2016;150:415-25. [Crossref] [PubMed]
- Aliberti S, Di Pasquale M, Zanaboni AM, et al. Stratifying risk factors for multidrug-resistant pathogens in hospitalized patients coming from the community with pneumonia. Clin Infect Dis 2012;54:470-8. [Crossref] [PubMed]
- Karhu J, Ala-Kokko TI, Vuorinen T, et al. Lower respiratory tract virus findings in mechanically ventilated patients with severe community-acquired pneumonia. Clin Infect Dis 2014;59:62-70. [Crossref] [PubMed]
- Wu X, Wang Q, Wang M. Set al. Incidence of respiratory viral infections detected by PCR and real-time PCR in adult patients with community-acquired pneumonia: a meta-analysis. Respiration 2015;89:343-52. [Crossref] [PubMed]
- Wiemken T, Peyrani P, Bryant K, et al. Incidence of respiratory viruses in patients with community-acquired pneumonia admitted to the intensive care unit: results from the Severe Influenza Pneumonia Surveillance (SIPS) project. Eur J Clin Microbiol Infect Dis 2013;32:705-10. [Crossref] [PubMed]
- Garg S, Jain S, Dawood FS, et al. Pneumonia among adults hospitalized with laboratory-confirmed seasonal influenza virus infection-United States, 2005-2008. BMC Infect Dis 2015;15:369. [Crossref] [PubMed]
- Piralla A, Rovida F, Girello A, et al. Frequency of respiratory virus infections and next-generation analysis of influenza A/H1N1pdm09 dynamics in the lower respiratory tract of patients admitted to the ICU. PloS One 2017;12:e0178926. [Crossref] [PubMed]
- Shah RD, Wunderink RG. Viral Pneumonia and Acute Respiratory Distress Syndrome. Clin Chest Med 2017;38:113-25. [Crossref] [PubMed]
- Ramsey C, Kumar A. H1N1: viral pneumonia as a cause of acute respiratory distress syndrome. Curr Opin Crit Care 2011;17:64-71. [Crossref] [PubMed]
- Scheltema NM, Gentile A, Lucion F, et al. Global respiratory syncytial virus-associated mortality in young children (RSV GOLD): a retrospective case series. Lancet Glob Health 2017;5:e984-91. [Crossref] [PubMed]
- Nair H, Nokes DJ, Gessner BD, et al. Global burden of acute lower respiratory infections due to respiratory syncytial virus in young children: a systematic review and meta-analysis. Lancet 2010;375:1545-55. [Crossref] [PubMed]
- Lee N, Qureshi ST. Other viral pneumonias: coronavirus, respiratory syncytial virus, adenovirus, hantavirus. Crit Care Clin 2013;29:1045-68. [Crossref] [PubMed]
- Riquelme R, Torres A, Rioseco ML, et al. Influenza pneumonia: a comparison between seasonal influenza virus and the H1N1 pandemic. Eur Respir J 2011;38:106-11. [Crossref] [PubMed]
- van den Hoogen BG, de Jong JC, et al. A newly discovered human pneumovirus isolated from young children with respiratory tract disease. Nat Med 2001;7:719-24. [Crossref] [PubMed]
- Holmes KV. SARS-associated coronavirus. N Engl J Med 2003;348:1948-51. [Crossref] [PubMed]
- Allander T, Tammi MT, Eriksson M, et al. Cloning of a human parvovirus by molecular screening of respiratory tract samples. Proc Natl Acad Sci U S A 2005;102:12891-6. [Crossref] [PubMed]
- Cauchemez S, Fraser C, Van Kerkhove MD, et al. Middle East respiratory syndrome coronavirus: quantification of the extent of the epidemic, surveillance biases, and transmissibility. Lancet Infect Dis 2014;14:50-6. [Crossref] [PubMed]
- Alimi Y, Lim WS, Lansbury L, et al. Systematic review of respiratory viral pathogens identified in adults with community-acquired pneumonia in Europe. J Clin Virol 2017;95:26-35. [Crossref] [PubMed]
- Dickson RP, Erb-Downward JR, Martinez FJ, et al. The Microbiome and the Respiratory Tract. Annu Rev Physiol 2016;78:481-504. [Crossref] [PubMed]
- Huffnagle GB, Dickson RP, Lukacs NW. The respiratory tract microbiome and lung inflammation: a two-way street. Mucosal Immunol 2017;10:299-306. [Crossref] [PubMed]
- Abeles SR, Jones MB, Santiago-Rodriguez TM, et al. Microbial diversity in individuals and their household contacts following typical antibiotic courses. Microbiome 2016;4:39. [Crossref] [PubMed]
- Chen C, Shen T, Tian F, et al. New microbiota found in sputum from patients with community-acquired pneumonia. Acta Biochim Biophys Sin (Shanghai) 2013;45:1039-48. [Crossref] [PubMed]
Cite this article as: Cillóniz C, Cardozo C, García-Vidal C. Epidemiology, pathophysiology, and microbiology of community-acquired pneumonia. Ann Res Hosp 2018;2:1.