Endothelial dysfunction in HIV infection: experimental and clinical evidence on the role of oxidative stress
The face of the human immunodeficiency virus/acquired immune deficiency syndrome (HIV/AIDS) epidemic in the developed world has undergone major shifts since the introduction of anti-retroviral therapy (ART) and subsequent development of advanced regimens and new drug classes. This has transformed the disease from a near-fatal diagnosis to a chronic infection that can be managed medically with minimal adverse effects or impact on the quality of life of the HIV+ individual. Indeed, individuals diagnosed with HIV now are expected to have a near-normal or normal life expectancy compared to their seronegative counterparts (1). This increased life expectancy invariably means that general medical conditions associated with ageing, including coronary artery disease (CAD), will increasingly be forming a part of integrated routine HIV care. Indeed, in a 2011 study (2), cardiovascular disease was found to be responsible for 8–22% of non-AIDS related causes of death in HIV+ individuals, with a proportionate increase of risk as age progresses.
Impaired endothelial function is one of the early stages of the atherosclerotic process. Several studies have shown that HIV infection is associated with endothelial dysfunction, with multiple mechanisms identified. Indeed, presence of HIV infection has been shown to independently confer increased risk for acute coronary syndromes and CAD compared to the general population (3), with an incidence of 3.88 per 1,000 patient-years in HIV+ individuals compared to 2.21 in seronegative subjects. A 2012 meta-analysis (4) reported that the relative risk of developing CAD in HIV positive individuals was ~61% higher than the seronegative population. Given the progressive, chronic nature of the atherosclerotic plaque formation process, this has important clinical implications for people living with HIV, especially in the context of the vastly increased life expectancy for these individuals, as mentioned previously.
The following review will focus on the clinical and molecular evidence available for the role of oxidative stress in the pathophysiology of HIV-induced endothelial dysfunction.
Endothelial dysfunction and oxidative stress in the vascular wall
Endothelial dysfunction is defined as impaired endothelium-dependent vasorelaxation in response to stimuli that normally trigger vasodilation. It is widely regarded as one of the first steps in the formation of the atherosclerotic plaque and other cardiovascular disease-states. On a molecular level, endothelial dysfunction is characterised by reduced vascular bioavailability of nitric oxide (NO). NO is a gaseous molecule which diffuses to the vascular smooth muscle cells, triggers an increase in intracellular cyclic GMP and induces vasorelaxation. It has a major role in the regulation of vascular tone, as it opposes the actions of vasoconstricting factors such as endothelin-1. Moreover, NO is also implicated in the regulation of other vascular processes, such as platelet aggregation and leukocyte adhesion, thus playing an integral role in vascular homeostasis (5).
Increased oxidative stress is one of the key causes of endothelial dysfunction. Oxidative stress is defined as the imbalance between the production of reactive oxygen species (ROS) and the endogenous antioxidant mechanisms to counteract the effects of ROS or to repair the resulting damage (6). ROS are responsible for direct damage of cellular structures within the vascular wall and are also capable of triggering a number of redox sensitive transcriptional pathways.
Under physiological conditions, several tightly controlled oxidative pathways contribute towards ROS production, while several intra- and extra-cellular antioxidant enzymatic mechanisms account for ROS elimination (6). There are two main enzymatic systems responsible for ROS generation in the vasculature: nicotinamide adenine dinucleotide phosphate (NADPH)-oxidases and nitric oxide synthases (NOS), with the endothelial NOS (eNOS) being the most important in the vascular wall, the others being iNOS (inducible) and nNOS (neuronal).
NADPH-oxidases were first characterized in phagocytic cells, where they play a pivotal role in host defense by generating superoxide radicals (O2·-) through the transfer of electrons from NADPH to O2 (7). The phagocytic NADPH-oxidase consists of two membrane-bound subunits: p22phox and gp91phox (together comprising the cytochrome b558 complex), and four cytosolic subunits: p40phox, p47phox, p67phox and a small GTP-binding protein (Rac1 or 2), which upon stimulation translocates to flavocytochrome b558 and activates the enzymatic complex. Several distinct NADPH-oxidases have been described in various cells, based on the presence of different homologues of the gp91phox membrane subunit (Figure 1). Human cardiovascular cells express NOX1, NOX2 (which is the homologue of phagocyte NADPH-oxidase), NOX4 and NOX5 (8). However, this expression profile varies considerably in different cells of the vascular wall, as well as in different vessels. More specifically, endothelial cells (ECs) have been shown to express all NOX isoforms, but mainly NOX2 and NOX4, with NOX2 being the most abundant isoform in human vein endothelium (9). In vascular smooth muscle cells, NOX4 is the most abundant NOX isoform of NADPH-oxidases, located mainly in the medial layer of human arteries (7), with NOX1 also expressed in lower amounts. Human cardiac tissue mainly expresses NOX2 and NOX4 (10). The vascular isoforms of NADPH-oxidases are constitutively active, generating a low but steady amount of O2·-, the production rate of which is ~1–10% of that in phagocytes (8). This rate can be increased by a number of agonists and stimuli, such as angiotensin II, thrombin, tumor necrosis factor-α (TNFα), interleukin-1 (IL-1), vascular endothelial growth factor (VEGF) or mechanical forces like shear stress. The constitutive and rather low activity of vascular NADPH-oxidases suggests that, under physiological conditions, they are involved in physiological vascular redox signaling. In disease states however, it is well-established that elevated expression and activity of NADPH-oxidases isoforms in the vasculature, together with the resulting increased ROS production, contribute to the initiation and maintenance of the atherosclerotic process.
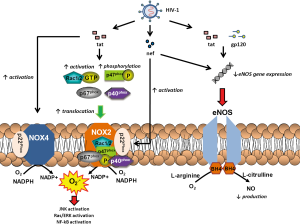
The second most important source of ROS in the vasculature is eNOS which is located predominantly in ECs. ENOS is a complex homodimer that uses L-arginine and molecular O2 as substrate to produce NO and L-citrulline. This is achieved through the transfer of electrons from the flavins of the reductase domain on one monodimer to the oxidase domain of the other, where the iron-containing heme active site is located (7). The presence of calmodulin, which is activated by calcium-binding, increases the rate of electron flow. At the heme site, the electrons are utilized to reduce and activate O2, which is in turn used to produce NO through oxidation of L-arginine. This process requires the binding of the essential co-factor tetrahydrobiopterin (BH4). When BH4 is bound to eNOS, the enzyme is considered to be “coupled” (11). Coupling of eNOS is essential for the physiological function of the human vessel. Degradation of BH4 by ROS, especially peroxynitrite (ONOO−), leads to what is known as “uncoupling” of the enzyme: in the absence of BH4, the flow of electrons within eNOS is disturbed; this leads to dissociation of the ferrous-dioxygen complex, causing eNOS to convert O2 into O2·− instead of producing NO (12). O2·− reacts with NO to form ONOO−, which can further oxidize BH4, creating a vicious cycle of eNOS uncoupling. The resulting drop in production of NO inhibits endothelium-dependent vasorelaxation, disturbs vascular homeostasis and leads to endothelial dysfunction (11). In addition, ONOO− has well-established roles in low density lipoprotein (LDL) oxidation and is also responsible for the nitration of various cellular components; accumulation of nitrated proteins is a potent marker of oxidative/nitrosative cellular damage. ONOO− can induce cellular apoptosis and even necrosis at high concentrations, further aggravating endothelial dysfunction (13).
Due to constant exposure to ROS generated through various pathways, almost all living cells possess several enzymatic antioxidant defense systems, controlling the final ROS availability (14). Superoxide dismutase (SOD) is an enzyme catalyzing the conversion of O2·− to hydrogen peroxide (H2O2). There are three SOD isoforms in human cells: SOD1 is located in the cytoplasm, SOD2 in the mitochondria and SOD3 is extracellular. Catalase is an enzyme located in peroxisomes and decomposes H2O2 to water and O2. Glutathione (GSH) is a tripeptide that acts as an important antioxidant limiting the effects of H2O2; this is achieved by reducing sulfhydryl groups in cysteine residues of other proteins, thus protecting those proteins of oxidative damage. This is catalyzed by glutathione-S-transferases, as well as glutathione peroxidases (GSH-Px), leading to the formation of glutathione disulfide (GSSG) from two GSH molecules; GSH can be replenished through the effects of glutathione reductase. The GSH/GSSG ratio is widely used as a marker of cellular redox state. Paraoxonase is an enzyme involved in protection against oxidation of the LDL molecule. Other antioxidant enzymatic systems are also present in cells, such as thioredoxins, peroxiredoxins, etc.
Clinical evidence for endothelial dysfunction in HIV+ individuals
Early studies before the advent of ART had shown that HIV infection is associated with serious cardiovascular abnormalities. Post-mortem examinations of children and young patients who died of AIDS revealed a vast array of coronary artery pathologies, from a vasculitis-like picture characterised by vascular wall infiltration by mononuclear cells and lymphocytes (15) to advanced atherosclerotic lesions disproportionate to the subject’s age (16). Other vascular pathologies seen in HIV patients are medium and large-artery aneurysms (17) as well as small-vessel vasculitides (18) in untreated individuals. These studies first gave evidence for harmful effects of HIV infection on cardiovascular function.
A non-invasive method of assessment of endothelial function is flow-mediated dilatation (FMD) of the brachial artery (19). This technique utilises ultrasound to measure the flow-mediated change in brachial artery diameter after an ischaemic challenge; this is induced by shear-stress which leads to endothelium-dependent vasodilation via increased production of NO from eNOS. The same technique can measure endothelium-independent vasodilation after administration of glyceryl trinitrite spray. Another commonly measured marker that can be easily assessed with ultrasound is carotid intima-media thickness (cIMT), which is an early indication of carotid atherosclerotic disease (20).
Multiple studies have examined the relationship between HIV infection and endothelial function in a clinical setting. Individuals with higher HIV-1 viral load show an inverse association with endothelium-dependent FMD (21). In a larger study of 75 HIV-1 infected individuals and 223 controls, FMD was significantly reduced in seropositive individuals and viral load was an independent predictor of the degree of reduction (22). A case-control study of HIV+ children, some taking ART and some not, concluded that both FMD and cIMT were significantly increased in seropositive children compared to controls, although treatment with a protease inhibitor was also an independent risk factor for endothelial dysfunction (23). Similar results were obtained in another study which showed that HIV-1-infected children, when compared to seronegative and ART-treated counterparts, demonstrated increased arterial wall stiffness, impaired endothelial function measured by FMD and reduced distensibility of the carotids (24). In a large 2008 case-control study of almost 1,500 subjects, presence of HIV-infection and treatment with ART were found to be independent predictors of early carotid atherosclerosis, with an increased cardiovascular risk in the range of 4–14% (25). Indeed, most research into the association of HIV infection and endothelial function that has been performed so far has been small case-control studies. A multi-centre, longitudinal study of cardiovascular risk and endothelial function in people living with HIV/AIDS (EndoAfrica study) is currently underway in South Africa (26); this study will allow a comprehensive assessment of cardiovascular risk and endothelial dysfunction in people living with HIV (Table 1).
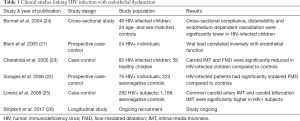
Full table
Table 1 summarises the currently available clinical studies linking HIV infection and endothelial dysfunction.
HIV structure and proteins
HIV can be divided into two major subtypes, HIV-1 and HIV-2. HIV-1 is the most widespread form of the virus, responsible for the HIV pandemic. The HIV-1 genome comprises nine main genes. Gag (group specific antigen) encodes for several structural proteins; pol (polymerase) encodes for the viral enzymes reverse transcriptase (RT) and RNase H, integrase and HIV protease; env encodes for the envelope proteins gp160, which is post-translationally cleaved into gp120 and gp41; vpu, vpr, vif and nef encode for accessory regulatory proteins and tat and rev encode for essential regulatory elements.
HIV tat (trans-activator of viral replication) increases transcription of the viral double stranded DNA and increases transcription of all HIV genes including its own gene, acting in a positive-feedback loop. It is secreted by infected cells and can penetrate other non-infected cells that are not typically a target for HIV where it can exert various deleterious effects (27). HIV nef (negative regulatory factor) is a protein which, although not necessary for HIV-1 entry and replication, dramatically increases HIV-1 viral load by enabling the virus to evade host immune response while at the same time increasing the amount of active T-cells available for the virus to infect (28). Both HIV tat and nef have been implicated in the pathogenesis of HIV-1 induced endothelial dysfunction (29); this will be discussed extensively in the following paragraphs.
ECs as a target for HIV-1
HIV-1 demonstrates a narrow tropism for specific cell types, mainly related to the cell surface receptor signature characteristic for each cell. More specifically, CD4 (cluster of differentiation 4) and co-receptors are usually necessary to enable effective infection of the cell by HIV-1. CCR5 (C-C chemokine receptor type 5) is the main co-receptor used in vivo; additionally, variants of the virus using CXCR4 (C-X-C chemokine receptor type 4) as co-receptor evolve during the course of the disease. In vitro, a large number of different co-receptors have been demonstrated to enable infection of various cell lines by HIV-1 strains (30). HIV-1 can also interact with other cell surface receptors through gp120 binding to galactocerebroside and its sulphated derivative (30).
The ability of HIV-1 to use a large number of different co-receptors led to the hypothesis that HIV-1 could potentially also infect ECs, which lack the main CCR5 and CXCR4 receptors. Indeed, HIV-1 demonstrates the in vitro ability to infect ECs, mostly dependent on the functional status of the cell, as the well as the tissue they originated from. Microvascular ECs derived from bone marrow, glomeruli, brain and liver can all be infected by HIV-1 without evidence of cytolysis (31,32). The ability of HIV-1 to infect brain endothelium is important in penetration of the blood-brain barrier and viral invasion of the central nervous system in vitro and in vivo (33). On the other hand, ECs derived from larger vessels are generally resistant to infection, except when exposed to a pro-inflammatory environment. In vitro data demonstrate that enhanced expression of intracellular adhesion molecule-1, mediated by RANTES (regulated on activation, normal T cell expressed and secreted) and interferon-γ, allows enhanced adhesion of HIV-1 infected T-cells on the surface of ECs (34,35), whereas other pro-inflammatory stimuli such as TNFα and IL-1 enable viral replication in macrovascular ECs (34). HIV-1 proteins have been directly associated with endothelial dysfunction and atherosclerosis-related vascular changes in animal models: in an HIV-1-transgenic mouse model (a non-infectious model containing the genetic sequences for env, tat, nef, rev, vif, vpr and vpu, but without gag and pol, therefore unable to cause replicating infection), aortic rings show significant endothelial dysfunction (36). However, in vivo measurements have not been able to show presence of replicating HIV-1 in ECs. When considering the mechanisms of endothelial dysfunction in the context of HIV-1, current evidence would suggest that it is not direct infection of HIV-1 cells responsible, but rather the ability of HIV-1 infection to trigger a pro-oxidant, pro-inflammatory microenvironment in the vascular wall.
HIV and systemic/vascular oxidative stress
Multiple studies have shown evidence of increased systemic oxidative stress in the context of HIV infection, as demonstrated by increased levels of circulating oxidative stress biomarkers. HIV-1-positive individuals exhibit higher circulating levels of malondialdehyde (MDA) and hydroperoxides compared with seronegative controls (37). In a recent nested case-control study of 54 HIV-infected individuals and 93 controls, HIV infection was associated with increased plasma levels of F2-isoprostanes (F2-IsoPs) and MDA; importantly, F2-IsoPs levels were also independently associated with all-cause mortality (38).
In addition to systemic oxidative stress, HIV infection has been shown to directly affect the redox balance of the vascular wall itself. Sections of cardiac microvascular endothelium obtained from HIV-1-infected individuals with cardiovascular disease display markedly elevated 3-nitrotyrosine staining (a marker of peroxynitrite damage) compared to samples obtained from HIV-1-positive/cardiovascular disease-free patients or noninfected/cardiovascular disease-positive subjects (39). The following paragraphs will discuss currently available evidence on how HIV infection affects the various enzymatic sources of ROS in the vasculature.
Effects of HIV infection on NADPH-oxidases
One well-studied mechanism via which HIV affects oxidative stress in the vascular wall is the interaction between the HIV protein tat and NADPH-oxidases. Tat protein can efficiently enter a wide array of cells both in vitro and in vivo. Tat induces actin cytoskeletal rearrangements in human ECs through PAK1 (p21-activated kinase-1) and downstream activation of NADPH-oxidases, by inducing phosphorylation of the p47phox subunit and increasing superoxide generation. An important study by Wu et al. (40) showed that HIV-1 tat activates Rac1, Ras and Rho GTPases (Guanosine Triphosphate) in ECs. As mentioned previously, Rac1 is crucial for the activation of different isoforms of NADPH-oxidases. By knocking down Nox2 and Nox4 Rac1 in human umbilical vein ECs, the researchers showed that Rac1 bifurcates tat signalling, leading to simultaneous but distinct Nox2-dependent JNK (c-Jun N-terminal kinases) activation and Nox4-dependent Ras/ERK (extracellular signal-regulated kinases) activation (40). Simultaneous exposure to HIV tat and cocaine aggravates tight junction protein disruption and increases permeability of human pulmonary artery ECs, effects mediated by Nox2-induced activation of the Ras/Raf/ERK1/2 pathway (41).
Aside from endothelial function, a large number of studies have focused on the role of NADPH-oxidases stimulation by HIV tat in the development of AIDS-related neurological manifestations, such as dementia and encephalitis. A study using both pharmacological and siRNA-mediated Nox2 inhibition showed that the mechanism via which HIV-1 tat induces the expression of cell adhesion molecules ICAM-1 (intercellular adhesion molecule 1) and VCAM-1 (vascular cell adhesion molecule 1) in activated microglia and astrocytes is Nox2-dependent (42). Indeed, tat is able to induce significantly pro-inflammatory responses in microglia and macrophages via NADPH-oxidases-dependent mechanisms (43), leading to increase production of pro-inflammatory molecules such as interleukin-6 (IL-6), TNFα and monocyte chemoattractant protein 1 (MCP-1) and increased microglial-mediated neurotoxicity. Another mechanism via which HIV tat causes increased inflammatory response in astrocytes is a cross-talk between Nox2 and Histone deacetylase 6 (HDAC6) (44), which was found to be alleviated by hindsiipropane B (45).
In addition to tat, an elegant 2014 study by Wang et al. (46) showed that the HIV protein nef also causes endothelial dysfunction by activating Nox2 and leading to increased ROS formation. This directly causes increased endothelial cell apoptosis and is associated with activation of the ROS-sensitive pro-inflammatory pathway of NF-Kb (nuclear factor kappa-light-chain-enhancer of activated B cells), causing increased MCP-1 production. Importantly, the researchers demonstrated in vivo relevance of these results by showing that HIV-infected T-cells can directly transfer nef protein into the endothelium (46).
Aside from HIV infection itself, some antiretroviral medications have been shown to exert adverse cardiovascular effects through actions on ROS-generating enzymes in the vasculature. For example, ritonavir has been shown to increase basal and NADPH-stimulated O2·− generation in porcine arteries as measured by lucigenin-enhanced chemiluminescence and visualised by DHE and nitrotyrosine staining (47). This was associated with impaired vasorelaxation of porcine artery rings, suggesting that the increase in ROS mainly mediated via NADPH-oxidases causes endothelial dysfunction.
Effects of HIV infection on NOS
Various HIV-1 proteins have been shown to exert adverse effects on endothelial function by affecting eNOS activity and expression. A seminal study by Paladugu et al. (48) showed that the HIV protein tat causes reduced endothelium-dependent vasodilation of porcine coronary arteries; this is in part mediated by tat-induced downregulation of eNOS mRNA, with subsequent reduction in eNOS protein levels. On the other hand, in human brain microvascular ECs, HIV-1 tat led to an increase in NO production via up-regulation of eNOS and iNOS; however, this increased NO production was linked to increased apoptosis in these cells, an effect prevented by NOS inhibitors (49). This would suggest that increased nitrosative stress induced by HIV-1 tat can cause adverse effects in the microvasculature. In addition to tat, the HIV protein nef has been shown to induce endothelial dysfunction in porcine pulmonary arteries, mediated at least in part by down-regulation of eNOS expression, reduced NO formation and increased O2·− production (50). These effects were prevented by Mn (III) tetrakis porphyrin, a SOD mimic (50). Moreover, gp120 has been shown to cause endothelial dysfunction in TNFα-activated porcine and human ECs, by reducing eNOS gene and protein expression levels (51). A schematic representation of the various mechanisms via which HIV-1 infection dysregulates ROS-generating enzymes in the vasculature to trigger endothelial dysfunction can be found in Figure 1.
Similar to effects on NADPH-oxidases, treatment with protease inhibitors has been shown to be linked to endothelial dysfunction via eNOS-mediated mechanisms. Treatment with ritonavir induces both endothelium dependent- and independent vascular dysfunction in porcine coronary arteries, via downregulation of eNOS and increased O2·− production (52); these effects were prevented by exposure to ginsenosides (53) and curcumin (54). The same group demonstrated similar effects for the protease inhibitors amprenavir and saquinavir (55).
Effects of HIV infection on antioxidant enzymes
Early studies had shown that HIV-1 proteins display the ability to suppress endogenous antioxidant enzymatic mechanisms. For example, HIV tat protein was shown to repress expression of manganese SOD in HeLa cells (56), whereas systemic levels of glutathione are significantly reduced in symptom-free seropositive individuals compared to seronegative controls (57). In terms of the relevance of these findings to endothelial dysfunction, an important study by Kline et al. (58) using an HIV-1 transgenic mouse model showed that, in this model, HIV infection was associated with impaired endothelial function and increased oxidative and nitrosative vascular stress. However, this was not related to increased NADPH-oxidases activity, reduced eNOS levels/activity or increased uncoupling. Rather, it was the result of decreased SOD and glutathione; as such, the researchers showed that the adverse cardiovascular effects observed in the transgene animals were reversed by administration of the glutathione precursor procysteine (58).
Several clinical studies have shown beneficial cardiovascular effects of dietary antioxidant supplementation in people living with HIV. Oral supplementation with vitamin C & E at the same time leads to a significant reduction in plasma lipid oxidation and other markers of oxidative stress in HIV-1-infected individuals (59). Multivitamin (B complex, vitamin C and E) supplementation is linked to reduced incidence of gestational hypertension in HIV-positive women (60). In a small randomized placebo-controlled clinical trial, supplementation of either β-carotene or selenium prevented an increase in circulating markers of endothelial dysfunction after one year of follow-up (61). However, in the general, seronegative population, the results of oral antioxidant supplementation in the prevention of cardiovascular disease have been largely disappointing, with a Cochrane meta-analysis of 2012 showing no beneficial effects on incidence of cardiovascular disease (62).
HIV and endothelial progenitor cells (EPCs)
One of the repair mechanisms of damaged vascular endothelium is mediated by the action of EPCs (63). EPC levels in the circulation are one of the markers of endothelial dysfunction and demonstrate good predictive value for vascular disease (63). HIV-1-seropositive patients have lower EPC levels than seronegative individuals (64). HIV-1 is able to directly infect of these cells, because they express CCR5 and CXCR4 on their cell surface, thus leading to a reduction in their number in the circulation; as such, antiretroviral therapy has been shown to restore EPC levels (65) by directly suppressing the virus.
Conclusions
Mounting clinical evidence suggests that HIV-1 infection is linked to increased incidence of cardiovascular disease, an effect which will only become more pronounced as time elapses and the population of people living with HIV grows older. In vitro data, animal studies and clinical data show that HIV-1 and certain HIV-protease inhibitors lead to endothelial dysfunction via a number of different mechanisms related to oxidative stress imbalance in the vasculature wall. The interdependence of these mechanisms is a matter of significant complexity, underpinning the need for better experimental models. Research on primates would offer important insights as this would most closely resemble human disease; however issues regarding costs and logistics make primate research especially cumbersome and difficult to perform. On the other hand, clinical trials in human populations are not without their own challenges, such as recruitment difficulties, adherence to treatment and loss to follow-up. Nevertheless, it is becoming clear that more well-designed and adequately powered clinical trials are required to investigate the efficacy of antioxidant interventions on cardiovascular risk in people living with HIV, to better address the future needs of this population.
Acknowledgements
This work was funded by a National Institute for Health Research (NIHR) Academic Clinical Fellowship to M Margaritis.
Footnote
Conflicts of Interest: The author has no conflicts of interest to declare.
References
- Sabin CA. Do people with HIV infection have a normal life expectancy in the era of combination antiretroviral therapy? BMC Med 2013;11:251. [Crossref] [PubMed]
- Bedimo RJ, Westfall AO, Drechsler H, et al. Abacavir use and risk of acute myocardial infarction and cerebrovascular events in the highly active antiretroviral therapy era. Clin Infect Dis 2011;53:84-91. [Crossref] [PubMed]
- Durand M, Sheehy O, Baril JG, et al. Association between HIV infection, antiretroviral therapy, and risk of acute myocardial infarction: a cohort and nested case-control study using Quebec's public health insurance database. J Acquir Immune Defic Syndr 2011;57:245-53. [Crossref] [PubMed]
- Islam FM, Wu J, Jansson J, et al. Relative risk of cardiovascular disease among people living with HIV: a systematic review and meta-analysis. HIV Med 2012;13:453-68. [PubMed]
- Deanfield JE, Halcox JP, Rabelink TJ. Endothelial function and dysfunction: testing and clinical relevance. Circulation 2007;115:1285-95. [Crossref] [PubMed]
- Antoniades C, Antonopoulos AS, Bendall JK, et al. Targeting redox signaling in the vascular wall: from basic science to clinical practice. Curr Pharm Des 2009;15:329-42. [Crossref] [PubMed]
- Channon KM, Guzik TJ. Mechanisms of superoxide production in human blood vessels: relationship to endothelial dysfunction, clinical and genetic risk factors. J Physiol Pharmacol 2002;53:515-24. [PubMed]
- Lassègue B, Clempus RE. Vascular NAD(P)H oxidases: specific features, expression, and regulation. Am J Physiol Regul Integr Comp Physiol 2003;285:R277-97. [Crossref] [PubMed]
- Guzik TJ, Sadowski J, Kapelak B, et al. Systemic regulation of vascular NAD(P)H oxidase activity and nox isoform expression in human arteries and veins. Arterioscler Thromb Vasc Biol 2004;24:1614-20. [Crossref] [PubMed]
- Antonopoulos AS, Margaritis M, Verheule S, et al. Mutual Regulation of Epicardial Adipose Tissue and Myocardial Redox State by PPAR-gamma/Adiponectin Signalling. Circ Res 2016;118:842-55. [Crossref] [PubMed]
- Landmesser U, Hornig B, Drexler H. Endothelial function: a critical determinant in atherosclerosis? Circulation 2004;109:II27-33. [Crossref] [PubMed]
- Alp NJ, Mussa S, Khoo J, et al. Tetrahydrobiopterin-dependent preservation of nitric oxide-mediated endothelial function in diabetes by targeted transgenic GTP-cyclohydrolase I overexpression. J Clin Invest 2003;112:725-35. [Crossref] [PubMed]
- Pacher P, Beckman JS, Liaudet L. Nitric oxide and peroxynitrite in health and disease. Physiol Rev 2007;87:315-424. [Crossref] [PubMed]
- Antoniades C, Tousoulis D, Tentolouris C, et al. Oxidative stress, antioxidant vitamins, and atherosclerosis. From basic research to clinical practice. Herz 2003;28:628-38. [Crossref] [PubMed]
- Joshi VV, Pawel B, Connor E, et al. Arteriopathy in children with acquired immune deficiency syndrome. Pediatr Pathol 1987;7:261-75. [Crossref] [PubMed]
- Paton P, Tabib A, Loire R, et al. Coronary artery lesions and human immunodeficiency virus infection. Res Virol 1993;144:225-31. [Crossref] [PubMed]
- Maniker AH, Hunt CD. Cerebral aneurysm in the HIV patient: a report of six cases. Surg Neurol 1996;46:49-54. [Crossref] [PubMed]
- Cebrián M, Miro O, Font C, et al. HIV-related vasculitis. AIDS Patient Care STDS 1997;11:245-58. [Crossref] [PubMed]
- Antoniades C, Mussa S, Shirodaria C, et al. Relation of preoperative radial artery flow-mediated dilatation to nitric oxide bioavailability in radial artery grafts used in off-pump coronary artery bypass grafting. Am J Cardiol 2009;103:216-20. [Crossref] [PubMed]
- Tousoulis D, Antoniades C, Vlachopoulos C, et al. Flow mediated dilation and carotid intima media thickness: clinical markers or just research tools? Int J Cardiol 2013;163:226-8. [Crossref] [PubMed]
- Blum A, Hadas V, Burke M, et al. Viral load of the human immunodeficiency virus could be an independent risk factor for endothelial dysfunction. Clin Cardiol 2005;28:149-53. [Crossref] [PubMed]
- Solages A, Vita JA, Thornton DJ, et al. Endothelial function in HIV-infected persons. Clin Infect Dis 2006;42:1325-32. [Crossref] [PubMed]
- Charakida M, Donald AE, Green H, et al. Early structural and functional changes of the vasculature in HIV-infected children: impact of disease and antiretroviral therapy. Circulation 2005;112:103-9. [Crossref] [PubMed]
- Bonnet D, Aggoun Y, Szezepanski I, et al. Arterial stiffness and endothelial dysfunction in HIV-infected children. AIDS 2004;18:1037-41. [Crossref] [PubMed]
- Lorenz MW, Stephan C, Harmjanz A, et al. Both long-term HIV infection and highly active antiretroviral therapy are independent risk factors for early carotid atherosclerosis. Atherosclerosis 2008;196:720-6. [Crossref] [PubMed]
- Strijdom H, De Boever P, Walzl G, et al. Cardiovascular risk and endothelial function in people living with HIV/AIDS: design of the multi-site, longitudinal EndoAfrica study in the Western Cape Province of South Africa. BMC Infect Dis 2017;17:41. [Crossref] [PubMed]
- Li JC, Au KY, Fang JW, et al. HIV-1 trans-activator protein dysregulates IFN-gamma signaling and contributes to the suppression of autophagy induction. AIDS 2011;25:15-25. [Crossref] [PubMed]
- Joseph AM, Ladha JS, Mojamdar M, et al. Human immunodeficiency virus-1 Nef protein interacts with Tat and enhances HIV-1 gene expression. FEBS Lett 2003;548:37-42. [Crossref] [PubMed]
- Wang T, Yi R, Green LA, et al. Increased cardiovascular disease risk in the HIV-positive population on ART: potential role of HIV-Nef and Tat. Cardiovasc Pathol 2015;24:279-82. [Crossref] [PubMed]
- Clapham PR, McKnight A. HIV-1 receptors and cell tropism. Br Med Bull 2001;58:43-59. [Crossref] [PubMed]
- Moses AV, Bloom FE, Pauza CD, et al. Human immunodeficiency virus infection of human brain capillary endothelial cells occurs via a CD4/galactosylceramide-independent mechanism. Proc Natl Acad Sci U S A 1993;90:10474-8. [Crossref] [PubMed]
- Lafon ME, Gendrault JL, Royer C, et al. Human endothelial cells isolated from the hepatic sinusoids and the umbilical vein display a different permissiveness for HIV1. Res Virol 1993;144:99-104. [Crossref] [PubMed]
- Bagasra O, Lavi E, Bobroski L, et al. Cellular reservoirs of HIV-1 in the central nervous system of infected individuals: identification by the combination of in situ polymerase chain reaction and immunohistochemistry. AIDS 1996;10:573-85. [Crossref] [PubMed]
- Conaldi PG, Serra C, Dolei A, et al. Productive HIV-1 infection of human vascular endothelial cells requires cell proliferation and is stimulated by combined treatment with interleukin-1 beta plus tumor necrosis factor-alpha. J Med Virol 1995;47:355-63. [Crossref] [PubMed]
- Dianzani F, Scheglovitova O, Gentile M, et al. Interferon gamma stimulates cell-mediated transmission of HIV type 1 from abortively infected endothelial cells. AIDS Res Hum Retroviruses 1996;12:621-7. [Crossref] [PubMed]
- Hansen L, Parker I, Sutliff RL, et al. Endothelial dysfunction, arterial stiffening, and intima-media thickening in large arteries from HIV-1 transgenic mice. Ann Biomed Eng 2013;41:682-93. [Crossref] [PubMed]
- Favier A, Sappey C, Leclerc P, et al. Antioxidant status and lipid peroxidation in patients infected with HIV. Chem Biol Interact 1994;91:165-80. [Crossref] [PubMed]
- Masiá M, Padilla S, Fernandez M, et al. Oxidative Stress Predicts All-Cause Mortality in HIV-Infected Patients. PLoS One 2016;11:e0153456. [Crossref] [PubMed]
- Baliga RS, Chaves AA, Jing L, et al. AIDS-related vasculopathy: evidence for oxidative and inflammatory pathways in murine and human AIDS. Am J Physiol Heart Circ Physiol 2005;289:H1373-80. [Crossref] [PubMed]
- Wu RF, Ma Z, Myers DP, et al. HIV-1 Tat activates dual Nox pathways leading to independent activation of ERK and JNK MAP kinases. J Biol Chem 2007;282:37412-9. [Crossref] [PubMed]
- Dalvi P, Wang K, Mermis J, et al. HIV-1/cocaine induced oxidative stress disrupts tight junction protein-1 in human pulmonary microvascular endothelial cells: role of Ras/ERK1/2 pathway. PLoS One 2014;9:e85246. [Crossref] [PubMed]
- Song HY, Ju SM, Seo WY, et al. Nox2-based NADPH oxidase mediates HIV-1 Tat-induced up-regulation of VCAM-1/ICAM-1 and subsequent monocyte adhesion in human astrocytes. Free Radic Biol Med 2011;50:576-84. [Crossref] [PubMed]
- Turchan-Cholewo J, Dimayuga VM, Gupta S, et al. NADPH oxidase drives cytokine and neurotoxin release from microglia and macrophages in response to HIV-Tat. Antioxid Redox Signal 2009;11:193-204. [Crossref] [PubMed]
- Youn GS, Cho H, Kim D, et al. Crosstalk between HDAC6 and Nox2-based NADPH oxidase mediates HIV-1 Tat-induced pro-inflammatory responses in astrocytes. Redox Biol 2017;12:978-86. [Crossref] [PubMed]
- Jo H, Jang HY, Youn GS, et al. Hindsiipropane B alleviates HIV-1 Tat-induced inflammatory responses by suppressing HDAC6-NADPH oxidase-ROS axis in astrocytes. BMB Rep 2018;51:394-9. [Crossref] [PubMed]
- Wang T, Green LA, Gupta SK, et al. Transfer of intracellular HIV Nef to endothelium causes endothelial dysfunction. PLoS One 2014;9:e91063. [Crossref] [PubMed]
- Conklin BS, Fu W, Lin PH, et al. HIV protease inhibitor ritonavir decreases endothelium-dependent vasorelaxation and increases superoxide in porcine arteries. Cardiovasc Res 2004;63:168-75. [Crossref] [PubMed]
- Paladugu R, Fu W, Conklin BS, et al. Hiv Tat protein causes endothelial dysfunction in porcine coronary arteries. J Vasc Surg 2003;38:549-55; discussion 555-6. [Crossref] [PubMed]
- Kim TA, Avraham HK, Koh YH, et al. HIV-1 Tat-mediated apoptosis in human brain microvascular endothelial cells. J Immunol 2003;170:2629-37. [Crossref] [PubMed]
- Duffy P, Wang X, Lin PH, et al. HIV Nef protein causes endothelial dysfunction in porcine pulmonary arteries and human pulmonary artery endothelial cells. J Surg Res 2009;156:257-64. [Crossref] [PubMed]
- Jiang J, Fu W, Wang X, et al. HIV gp120 induces endothelial dysfunction in tumour necrosis factor-alpha-activated porcine and human endothelial cells. Cardiovasc Res 2010;87:366-74. [Crossref] [PubMed]
- Fu W, Chai H, Yao Q, et al. Effects of HIV protease inhibitor ritonavir on vasomotor function and endothelial nitric oxide synthase expression. J Acquir Immune Defic Syndr 2005;39:152-8. [PubMed]
- Chai H, Zhou W, Lin P, et al. Ginsenosides block HIV protease inhibitor ritonavir-induced vascular dysfunction of porcine coronary arteries. Am J Physiol Heart Circ Physiol 2005;288:H2965-71. [Crossref] [PubMed]
- Chai H, Yan S, Lin P, et al. Curcumin blocks HIV protease inhibitor ritonavir-induced vascular dysfunction in porcine coronary arteries. J Am Coll Surg 2005;200:820-30. [Crossref] [PubMed]
- Chai H, Yang H, Yan S, et al. Effects of 5 HIV protease inhibitors on vasomotor function and superoxide anion production in porcine coronary arteries. J Acquir Immune Defic Syndr 2005;40:12-9. [Crossref] [PubMed]
- Flores SC, Marecki JC, Harper KP, et al. Tat protein of human immunodeficiency virus type 1 represses expression of manganese superoxide dismutase in HeLa cells. Proc Natl Acad Sci U S A 1993;90:7632-6. [Crossref] [PubMed]
- Buhl R, Jaffe HA, Holroyd KJ, et al. Systemic glutathione deficiency in symptom-free HIV-seropositive individuals. Lancet 1989;2:1294-8. [Crossref] [PubMed]
- Kline ER, Kleinhenz DJ, Liang B, et al. Vascular oxidative stress and nitric oxide depletion in HIV-1 transgenic rats are reversed by glutathione restoration. Am J Physiol Heart Circ Physiol 2008;294:H2792-804. [Crossref] [PubMed]
- Allard JP, Aghdassi E, Chau J, et al. Oxidative stress and plasma antioxidant micronutrients in humans with HIV infection. Am J Clin Nutr 1998;67:143-7. [Crossref] [PubMed]
- Merchant AT, Msamanga G, Villamor E, et al. Multivitamin supplementation of HIV-positive women during pregnancy reduces hypertension. J Nutr 2005;135:1776-81. [Crossref] [PubMed]
- Constans J, Seigneur M, Blann AD, et al. Effect of the antioxidants selenium and beta-carotene on HIV-related endothelium dysfunction. Thromb Haemost 1998;80:1015-7. [Crossref] [PubMed]
- Bjelakovic G, Nikolova D, Gluud LL, et al. Antioxidant supplements for prevention of mortality in healthy participants and patients with various diseases. Cochrane Database Syst Rev 2012.CD007176. [PubMed]
- Moreno PR, Sanz J, Fuster V. Promoting mechanisms of vascular health: circulating progenitor cells, angiogenesis, and reverse cholesterol transport. J Am Coll Cardiol 2009;53:2315-23. [Crossref] [PubMed]
- da Silva EF, Fonseca FA, Franca CN, et al. Imbalance between endothelial progenitors cells and microparticles in HIV-infected patients naive for antiretroviral therapy. AIDS 2011;25:1595-601. [Crossref] [PubMed]
- Teofili L, Iachininoto MG, Capodimonti S, et al. Endothelial progenitor cell trafficking in human immunodeficiency virus-infected persons. AIDS 2010;24:2443-50. [Crossref] [PubMed]
Cite this article as: Margaritis M. Endothelial dysfunction in HIV infection: experimental and clinical evidence on the role of oxidative stress. Ann Res Hosp 2019;3:7.