The role of insulin growth factors in autoimmune diseases
Introduction
The insulin-like growth factor (IGF) pathway includes IGF (IGF-I and IGF-II), insulin-like growth factor binding proteins (IGFBPs) and insulin-like growth factor receptors (IGF-IR and IGF-IIR) (1,2).
IGFs play key roles in normal growth, metabolism and homeostasis of organisms. Their main production site is in the liver under the control of growth hormone (GH). The detection of their differential expression in pathological conditions, such as cancer, cardiovascular and metabolic diseases, has stimulated researchers’ interest in designing new and promising therapeutic approaches (3,4).
Several recent studies reveal the interaction of IGF pathway with the immune system. IGFs’ detection at mRNA and protein level in peripheral blood mononuclear cells, the interaction of the growth factor pathway with various cytokines (e.g., interferons) and immune cells such as T lymphocytes, macrophages and bone marrow cells, are only few examples (5-7). Moreover, dysregulation of the IGFs pathway in the setting of various autoimmune diseases, such as type I diabetes, Grave’s disease, Crohn’s disease, rheumatoid arthritis (RA) and Sjogren’s syndrome (SS), implies a potential contributory role of IGFs in autoimmune pathogenesis (8-17).
The aim of the present review is to summarize the available literature regarding the role of IGF pathway in immune system regulation as well the pathophysiology of systemic autoimmune diseases.
IGF-1 gene: localization and isoforms
The human IGF-1 gene is located in the long arm of chromosome 12, contains six exons and gives rise to three mainly heterogeneous transcripts: Ea, Eb and Ec- through a combination of: (I) alternative use of promoters, (II) alternative splicing and (III) different polyadenylation signals (18,19).
These IGF-I transcripts encode three different peptides, which also undergo post-translational modification. IGF-I Ea transcript derives from the splicing pattern exon 1 or 2-3-4-6 of the IGF-1 gene represents the main IGF-I mRNA produced by the liver and other tissues as well, with similar exon sequence (20,21). IGF-I Eb transcript is a splice variant of exon 1 or 2-3-4-5. Its expression was initially detected in human liver (22), while it was also found to be expressed, in skeletal muscle and other various tissues and cells such as prostate and endometrium (19). IGF-I Ec transcript is an exon 1 or 2-3-4-5-6 splice variant of the IGF-1 gene. Structurally, the IGF-I Ec mRNA transcript differs from the IGF-I Ea variant by the presence of the first 49 base pairs from exon 5. IGF-I Ec mRNA transcript was initially identified in human liver, however, it is expressed at approximately 10% of the main IGF-I Ea transcript (21). This transcript was named mechano-growth factor (MGF) since it was found to be upregulated in response to muscle stretch and/or damage (23). Of interest, its expression has been also identified in various tissues such as endometrium (24), normal and cancerous prostatic cells (25), as well as in osteoblast-like osteosarcoma cells (26,27). Its regulation appears to be under IL-6 action (28).
The different IGF-I mRNA transcripts encode its precursor proteins, which differ in length of their amino-terminal peptide (signal peptide) and the structure of peptide E (extension peptide or E domain) at their final end. The last four (4) amino acids in the C region appear to be responsible for the strong affinity (binding) to the IGF-IR receptor and the transmission of its signal (19,29). The biological significance of splicing variants of the IGF-I gene is unknown and the molecular and physiological mechanisms regulating their expression are uncertain, however, these variants probably indicate the complexity of IGF-I activities through its different isoforms (18).
Insulin growth factor binding proteins and their biological role
IGF I and II are mainly produced by the liver under the action of GH which is secreted by the pituitary gland. The availability of IGF-I for binding to its receptor is determined by its interaction with the binding proteins. Six main types of insulin growth factor binding proteins (IGFBPs 1–6) has been identified which display very high affinity for IGF-I (30). The first five preferentially bind IGF-I over IGF-II, while IGFBP-6 has a 100-fold higher affinity for IGF-II compared to IGF-I (31,32). IGFBP-3 is the most abundant IGFBP in serum, is produced primarily from hepatocytes but is also produced from other tissues such as kidney, uterus and placenta (33).
In the circulation, more than 90% of both IGF-I and -II are bound to IGF binding proteins IGFBP3 or IGFBP5 and a glycoprotein called IGFALS. The rest are bound to the other IGFBPs and less than 1% circulates as a free form (34). IGF activity is determined by the ratio of free to bound IGF-I, since the bound form does not execute its biological activity (35). IGFBPs compete with IGF-IR and normally have higher binding affinity to IGF-I than IGF-IR does. Therefore, binding of IGFBPs to IGF-I prevents the ligand from interacting with the receptor and, therefore suppresses IGF-I actions. On the other hand, IGFBPs may act as a reservoir of slowly releasing IGF-I, preventing the receptor down regulation by the exposure to high IGF-Ι levels (36).
Another binding control mechanism is through post-translational modifications (ubiquitination, phosphorylation, etc.) that can alter their ability to bind to IGF-I (36). Post-translational modifications along with the presence of specific proteases that degrade IGFBPs/IGF-Ι complex have been associated with various types of cancer. There is also a group of cysteine-rich proteins, known as IGFBP-related proteins (IGFBP-rPs), that share important structural similarities with the IGFBPs, but they have low binding affinity to IGFs. It has been proposed that these proteins and the IGFBPs constitute an IGFBP superfamily, however, the functions of the IGFBP-rPs regarding the IGFs actions are yet unclear (37).
IGF receptors and downstream signaling
The IGF receptor (IGF-R) belongs to the family of transmembrane receptor tyrosine kinases and exhibits homology to the insulin receptor (IR). It includes two types: (I) type I, which is the major receptor of IGF-I and (II) type II, to which IGF-II is predominantly attached (38). Type I IGF-IR is composed of 1368 amino acids, consisting of two extracellular α-subunits, the IGF-I binding domain and two transmembrane β-subunits, which contain three tyrosine residues. IGF-I may also be associated with a lower affinity to both type II (IGF-IIR) and IR. The former primarily mediates the internalization of IGF-I as well as metabolic processes while the latter affects cell division (19).
A hybrid IGF-IR/IR receptor consisting of an IR semi-receptor that binds to an IGF-IR semi-receptor has been also described. IGF-Ι signaling through this hybrid receptor has been implicated in cancer biology (39).
The binding of IGF-I to α-subunits leads to phosphorylation of tyrosine residues which in turn activates a cascade of intracellular reactions leading to mitogenic, anti-apoptotic as well as cell differentiation actions by regulating both normal and abnormal cellular development. More specifically, cytoplasmic molecules incorporating insulin receptor substrate proteins (IRS) are activated (40), leading to initiation of two major signaling pathways (Figure 1); the first leads to the activation of 3-phosphatidylinositol kinase (PI3K) involved in cellular processes such as resistance to apoptosis (through protein kinase B or Akt activation), cellular metabolism and growth; the second includes proteins that are involved in the growth factor signaling pathway, such as growth factor receptor-bound protein 2 (Grb-2) and Src homology and Collagen (Shc). The latter is associated with Grb-2 which, through the Grb-SOS complex, promotes activation of Ras-Raf intermediates, which in turn activate extracellular signal–regulated kinases (ERK), leading to cell proliferation and growth. Ras-Raf activates mitogen-activated protein kinase kinase 1/2 (MKKs), resulting in phosphorylation and activation of extracellular-signal-regulated kinases 1/2 (ERK1/2), which then regulate the action of cellular and nuclear proteins, as well as transcription factors (41).
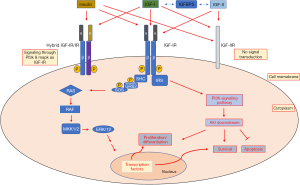
Expression of the IGF-Ι receptor seems to be dependent on the cell type, as well as the cellular microenvironment. Recent data reported significant overexpression in various cancer cells (42-44) as well as some autoimmune diseases (8).
The structure of the type II receptor consists of a single polypeptide chain without a kinase domain. It appears to be identical to the cation-independent mannose-6-phosphate receptor, to which it is attached. IGF-IIR does not have a signaling domain and is thought to be involved in the clearance of soluble IGF-II from serum and tissue fluids through receptor-mediated endocytosis (45).
The physiological action of insulin growth factors
IGFs are involved in many cell processes, including cell differentiation, cell growth, proliferation, and apoptosis (19).
IGF-I plays an essential role in body growth and metabolism, especially during the postnatal life, through the activation of IGF-IR. It has been suggested that serum IGF-I, which is postnatally produced mainly by the liver under GH stimulation though other tissues can serve as potential sources through autocrine and paracrine mechanisms (31). In contrast, serum IGF-I exerts a negative feedback on GH production, either through direct inhibition of the pituitary gland and or indirect stimulation of somatostatin (SST), a known inhibitor of growth hormone releasing hormone (GHRH) (31).
IGF-II is a circulating peptide hormone whose regulation can be under the control of GH (46). IGF-II plays an important role in both developmental and metabolic pathways, especially during the prenatal life. Indeed, IGF-II is preferentially expressed during embryogenesis and fetal development, it stimulates cell growth and proliferation and promotes embryo and fetus growth, through the activation of IGF-IR and IR, in particular the isoform A (IRA), which is predominantly expressed during prenatal life (39,47). IGF-II can also bind to IGF-IIR, which is considered a “scavenger receptor”. Indeed, IGF-II binding to IGF-IIR leads to IGF-II degradation by the lysosomes without eliciting proliferation or survival signals (48,49). Overall, IGFs may also play an autocrine or paracrine role by binding to IGF-IR and/or IR on target cells (50).
The interaction of insulin growth factors and immune system
Recent data highlight the interaction between GH, the IGF pathway and the immune system (51). For example, IGF-I levels decrease with age as well as in chronic inflammatory disorders status, resulting in poor immune responses. On the other hand, children with chronic inflammatory diseases exhibit a developmental disorder possibly through IGF-I/GH dysfunction as a result of excessive proinflammatory cytokines (52). IGF pathway has been shown to get involved in the growth of several immune cells including hematopoietic cells, B and T lymphocytes, macrophages and neutrophils (8). In experimental mouse model, hematopoiesis appears to increase after bone marrow transplantation and co-administration of IGF-I and/or GH (52). In bone marrow, administration of IGF-I promotes the production of mature B cells, while at the level of thymus and spleen it affects the growth of thymic and splenocyte cells, respectively. Depending on their degree of activation, T lymphocytes display differential expression of IGF-I, IGF-II, IGF-IR and IR (8,53). It has been further demonstrated that administration of IGF-I leads to cellular proliferation and chemotaxis possibly through interaction with a network of chemokines, whereas under other conditions it can lead to inhibition of T-cell growth by dampening IL-2 production (53-56). Increased expression of IGF-I is also observed in activated macrophages and neutrophils contributing to innate immune responses (57,58). Taken together, these data highlight an important role of GH/IGF-Ι pathway in immune functions, though the underlying mechanisms have not been fully elucidated.
The role of insulin growth factors on autoimmune diseases
Autoimmune diseases are a heterogeneous group of disorders with common etiopathogenetic mechanisms, but distinct clinical phenotypes. A genetically determined deregulated immune response in conjunction with hormonal and other environmental factors (e.g., stress, viruses, UV light, medications) leading to chronic inflammatory tissue damage is a well-accepted pathophysiological scenario in these disorders (59). In this context, the contribution of the IGF pathway in immune dysfunction appears to be a new field of investigation and potential target for therapeutic intervention.
RA
RA is a systemic chronic inflammatory disease of unclear etiology. It is characterized by immune activation, leucocyte infiltration and synovial inflammation untimely resulting in joint swelling (60). The cellular composition of the inflamed synovial fluid (SF) includes innate and adaptive immune cells such as T cells, B cells, monocytes and macrophages; moreover, fibroblasts promote chondrocyte catabolism and osteoclastogenesis resulting in articular destruction (61,62). The joint destruction is amplified by elevated levels of cytokines and GHs (63). The proliferation of synoviocytes leads to an increase in metabolic demands, which in turn fuels angiogenesis and expansion of new vessels into cartilage and bone, resulting in matrix degradation (64).
IGF-I is thought to have a key role in the maintenance of the steady-state metabolism of cartilage and several studies suggest that IGF-Ι stimulates the synthesis and decreases the degradation of proteoglycans in cultured cartilage explants (65,66).
The contribution of IGF/IGFBP axes in RA pathogenesis has been extensively examined giving controversial results. Several studies over the last years reported high IGFBP2, IGFBP3 and IGF-Ι levels in both plasma and SF of RA patients compared to controls (15,65,67-71), though there are works reporting similar or reduced IGF-I levels in SF (72-74) or reduced IGFBP3 serum levels in RA patients compared to controls (72,75,76). Recently, IGFBP6—a putative novel chemoattracting agent driving T-cell migration from the periphery to the inflamed joints in RA was also found upregulated in synovial RA tissue (77). Upregulation of IGFBPs and IGF-Ι have been also linked to the presence of proinflammatory cytokines such as interleukin-1 beta (IL-1β), tumor necrosis factor-alpha (TNFα) and C-reactive protein (CRP) (65,68). Of note, an important variant in the promoter of IGF1 gene has been previously shown to increase RA susceptibility, associated with low IGF-I serum levels and higher disease activity score (DAS) particularly in male individuals non-carrying the wild type 192-bp allele (78).
Additionally, the involvement of IGF-IR in disease pathogenesis has been recently revealed. The main finding is the increased IGF-IR expression in peripheral blood leukocytes which was associated with systemic inflammation (79,80). In contrast, in an arthritis mouse model reduced IGF-IR expression was detected in synovial tissue (ST) and restoration was achieved upon IL-27 and IL-35 treatment (81). Moreover, resistin suppression was associated with down-regulation of IGF-IR expression and a reduction in Akt phosphorylation in human synovial transplants in vivo. The observed high levels of resistin in RA SF were inversely correlated with IGF-I SF levels, while a clear IGF-IR expression was found in the synovium. Abrogation of resistin in the RA synovium using siRNA led to decreased phosphorylation of Akt, suggesting that resistin may activate Akt through IR/IGF-IR, facilitating excessive growth of ST in RA synovium (72).
Another key factor that regulates IGF-IR expression is micro RNA 223 (miR-223) which was found to be overexpressed in RA patients peripheral blood T cells (82-84) and inversely associated with IGF-IR expression (83).
Accumulating data so far, support the role of IGF-I/IGFBPs axes in human cachexia (31,54), a common manifestation among RA patients characterized by loss of fat-free mass, predominantly skeletal muscle (85). In an RA cohort of sixty patients was observed reduced serum IGF-Ι/IGFBP1 ratio in patients with cachexia (86). In line with these findings, decreased IGF-Ι and increased adiponectin serum levels were correlated with higher disease activity and lower muscle mass (87). Interestingly, high-intensity resistance exercise can reduce the RA related cachexia by increasing muscular IGF-Ι and IGFBP3 expression (88-90).
An interesting new study reported that the thioredoxin domain-containing 5 (TXNDC5) gene is associated with susceptibility to RA and exhibits increased expression in the STs. In vitro experiments showed that TXNDC5 gene expression contributes to abnormal RA synovial fibroblasts (RASFs) proliferation and migration by increasing IGF-I activity through reduction of IGFBP1 levels (91).
Juvenile idiopathic arthritis (JIA)
JIA is a systemic chronic inflammatory disease (92). The term describes a clinically heterogeneous group of arthritides of unknown cause, with onset prior to 16 years of age. Growth retardation is a common feature of JIA (93).
Several studies so far consistently revealed an association between low serum or plasma IGFBP3 and IGF-I levels with the stunted growth observed in the setting of JIA (94-103) (Table 1), possibly as a result of excessive inflammatory activity (97,102,104) or increased proteolytic activity of serum gelatinase (94), previously shown to mediate proteolytic degradation of both IGFBP3 and IGF-I (105,106). In contrast, no association between IGF-I levels and cartilage oligomeric matrix protein (COMP) was observed (97,102).
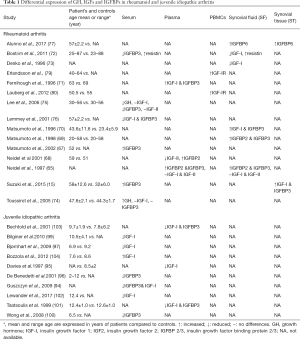
Full table
Interestingly, normalization of IGF-I and IGF-IBP3 levels was achieved following treatment with biological agents etanercept and tocilizumab resulting in growth reconstitution in JIA patients (107,108).
Systemic lupus erythematosus (SLE)
SLE is a severe, chronic autoimmune disorder characterized by involvement of multiple organ systems, loss of tolerance to self-antigens and dysregulated interferon responses. The disease can affect many different body systems, including joints, skin, kidneys, blood cells, heart, and lungs (109).
The clinical heterogeneity of SLE is accompanied by complex disturbances in the immune system, with the hallmark of characteristic autoantibodies and an enhanced type I interferon (IFN) and B-cell activating factor (BAFF)/B lymphocyte stimulator (BlyS) system (110).
The role of IGF/IGFBPs in SLE remains unclear. While no differences in IGF-I IGFBP3 or free IGF-I serum levels were observed in SLE patients compared to age-matched healthy controls (111,112), IGFBP2 serum levels - the second most prevalent serum IGFBP- have been found to be increased in patients with active SLE compared to healthy controls (113). Another study conducted in a cohort of 86 patients suffering from lupus nephritis (LN), a major complication of SLE, serum IGFBP4 were significantly higher and correlated with chronicity index of renal pathology in LN patients compared to controls (114). Moreover, high mRNA and protein expression of IGF-I and IGFBP2 in kidney sections (115) and increased IGFBP2 serum levels has been detected in a lupus mouse model (116) (Table 2).
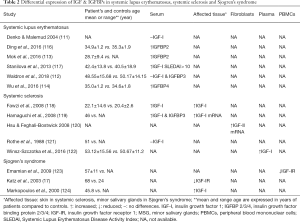
Full table
Of interest, a genetic variation +3179G/A of the IGF-1R gene has been recently shown to increase lupus susceptibility and severity in a Bulgarian population. Upon classification of these patients according to disease activity, significantly increased IGF-I serum levels were detected in very active SLE patients (Systemic Lupus Erythematosus Disease Index SLEDAI score > 10) compared to those with mild and moderate disease activity (SLEDAI ≤10) (117).
Systemic sclerosis (SSc)
SSc is a multiorgan disease, characterized by progressive fibrosis of the skin and internal organs (125). Alterations in immune system, vasculature and connective tissue are considered pathogenetic disease hallmarks (126).
IGF-I, among other growth factors, has been implicated in the pathogenesis of several fibrotic disorders (127). While initial reports did not detect any differences in IGF-I levels between SSc patients and controls (121), subsequent studies revealed increased IGF-I (118,119,122) and IGFBP3 serum levels and overexpression of IGF-I mRNA transcripts in skin biopsy of SSc patients (119) (Table 2). Moreover, following disease stratification, patients with diffuse cutaneous systemic sclerosis (dcSSc) displayed higher IGF-I levels compared to those with limited cutaneous systemic sclerosis (lcSSc) (119).
IGF-I/IGFBPs axis has been also implicated in the pathogenesis of pulmonary fibrosis which is the most common cause of SSc-related mortality (128). Increased IGF-I levels have been found in bronchoalveolar lavage fluid in patients with SSc-related pulmonary fibrosis as well as other forms of pulmonary fibrosis (129). In addition, immunohistochemical overexpression of IGF-II, in explanted lung tissues derived from SSc patients complicated by pulmonary fibrosis has been detected, as well as, increased IGF-II mRNA and protein expression in primary cultured fibroblasts derived from the same SSc lung tissues (120).
Sjögren’s syndrome (SS)
SS is a chronic autoimmune disorder that typically affects exocrine glands—mainly labial and lacrimal—leading to complaints of dry mouth and eyes. Given the presence of periepithelial mononuclear cell infiltrates, both in exocrine glands and in other parenchymal organs such as kidney, lung, and liver, the term “autoimmune epithelitis” has been proposed (130).
The presence of IGF-I in the saliva of mammals in association with immunohistochemical evidence of its expression in salivary glands both in human and in experimental models imply a contributory role for this molecule in salivary gland epithelium homeostasis (131). A recent study proposed that pre-treatment of mice with IGF-I before irradiation resulted in reduced DNA damage of salivary glands and alleviation of symptoms associated with radiation treatment (132). Of note, IGF-I expression decreases with age, providing an explanation for the salivary dysfunction related to the atrophic salivary epithelium found in the elderly population (133).
The contribution of the IGF pathway in maintaining both the number of cells of the salivary gland and intercellular junctions, and to ensure the functioning of the salivary glands suggest a possible involvement in the pathogenesis of SS (134). Limited data until today, have revealed reduced immunohistochemical detection of IGF-I receptor in experimental autoimmune sialadenitis (135) and salivary gland biopsies (17) along with increased IGF-I expression in salivary gland biopsies from SS patients compared to controls (124). Furthermore, microarray data on peripheral blood monocytes, showed a clear down-regulation of IGF-IR transcripts in SS patients compared to controls (123).
Conclusions
A growing body of evidence over the last years supports an important role of IGF/IGFBPs axis dysregulation in the pathogenesis of systemic rheumatic diseases. This can occur either through inhibitory growth signals leading eventually to apoptosis of host cells and generation of aberrant immune responses against self, or promotion of inflammatory processes mediated by inappropriate growth signals to selective immune and non-immune cell populations. Further research is required to fully elucidate the dual role of IGF/IGFBPs system in autoimmune pathology, possibly resulting in novel therapeutic targets.
Acknowledgements
None.
Footnote
Conflicts of Interest: The authors have no conflicts of interest to declare.
References
- Adams TE, Epa VC, Garrett TP, et al. Structure and function of the type 1 insulin-like growth factor receptor. Cell Mol Life Sci CMLS 2000;57:1050-93. [Crossref] [PubMed]
- Hjortebjerg R, Frystyk J. Determination of IGFs and their binding proteins. Best Pract Res Clin Endocrinol Metab 2013;27:771-81. [Crossref] [PubMed]
- Davison Z, de Blacquière GE, Westley BR, et al. Insulin-like growth factor-dependent proliferation and survival of triple-negative breast cancer cells: implications for therapy. Neoplasia 2011;13:504-15. [Crossref] [PubMed]
- Teppala S, Shankar A. Association between serum IGF-1 and diabetes among U.S. adults. Diabetes Care 2010;33:2257-9. [Crossref] [PubMed]
- Schillaci R, Brocardo MG, Galeano A, et al. Downregulation of insulin-like growth factor-1 receptor (IGF-1R) expression in human T lymphocyte activation. Cell Immunol 1998;183:157-61. [Crossref] [PubMed]
- Chen CY, Tseng KY, Lai YL, et al. Overexpression of Insulin-Like Growth Factor 1 Enhanced the Osteogenic Capability of Aging Bone Marrow Mesenchymal Stem Cells. Theranostics 2017;7:1598-611. [Crossref] [PubMed]
- van Buul-Offers SC, Kooijman R. The role of growth hormone and insulin-like growth factors in the immune system. Cell Mol Life Sci CMLS 1998;54:1083-94. [Crossref] [PubMed]
- Smith TJ. Insulin-like growth factor-I regulation of immune function: a potential therapeutic target in autoimmune diseases? Pharmacol Rev 2010;62:199-236. [Crossref] [PubMed]
- Raisingani M, Preneet B, Kohn B, et al. Skeletal growth and bone mineral acquisition in type 1 diabetic children; abnormalities of the GH/IGF-1 axis. Growth Horm IGF Res 2017;34:13-21. [Crossref] [PubMed]
- Nambam B, Schatz D. Growth hormone and insulin-like growth factor-I axis in type 1 diabetes. Growth Horm IGF Res 2018;38:49-52. [Crossref] [PubMed]
- Krieger CC, Perry JD, Morgan SJ, et al. TSH/IGF-1 Receptor Cross-Talk Rapidly Activates Extracellular Signal-Regulated Kinases in Multiple Cell Types. Endocrinology 2017;158:3676-83. [Crossref] [PubMed]
- Smith TJ, Janssen JAMJL. Insulin-like growth factor-I receptor and thyroid-associated ophthalmopathy. Endocr Rev 2019;40:236-67. [Crossref] [PubMed]
- Li C, Vu K, Hazelgrove K, et al. Increased IGF-IEc expression and mechano-growth factor production in intestinal muscle of fibrostenotic Crohn’s disease and smooth muscle hypertrophy. Am J Physiol Gastrointest Liver Physiol 2015;309:G888-899. [Crossref] [PubMed]
- DeBoer MD, Lee AM, Herbert K, et al. Increases in IGF-1 After Anti-TNF-α Therapy Are Associated with Bone and Muscle Accrual in Pediatric Crohn Disease. J Clin Endocrinol Metab 2018;103:936-45. [Crossref] [PubMed]
- Suzuki S, Morimoto S, Fujishiro M, et al. Inhibition of the insulin-like growth factor system is a potential therapy for rheumatoid arthritis. Autoimmunity 2015;48:251-8. [Crossref] [PubMed]
- Tsushima H, Morimoto S, Fujishiro M, et al. Kinase inhibitors of the IGF-1R as a potential therapeutic agent for rheumatoid arthritis. Autoimmunity 2017;50:329-35. [Crossref] [PubMed]
- Katz J, Stavropoulos F, Cohen D, et al. IGF-1 and insulin receptor expression in the minor salivary gland tissues of Sjögren’s syndrome and mucoceles - immunohistochemical study. Oral Dis 2003;9:7-13. [Crossref] [PubMed]
- Philippou A, Armakolas A, Koutsilieris M. Evidence for the Possible Biological Significance of the igf-1 Gene Alternative Splicing in Prostate Cancer. Front Endocrinol (Lausanne) 2013;4:31. [Crossref] [PubMed]
- Philippou A, Maridaki M, Pneumaticos S, et al. The Complexity of the IGF1 Gene Splicing, Posttranslational Modification and Bioactivity. Mol Med 2014;20:202-14. [Crossref] [PubMed]
- Jansen M, van Schaik FM, Ricker AT, et al. Sequence of cDNA encoding human insulin-like growth factor I precursor. Nature 1983;306:609-11. [Crossref] [PubMed]
- Chew SL, Lavender P, Clark AJ, et al. An alternatively spliced human insulin-like growth factor-I transcript with hepatic tissue expression that diverts away from the mitogenic IBE1 peptide. Endocrinology 1995;136:1939-44. [Crossref] [PubMed]
- Rotwein P. Two insulin-like growth factor I messenger RNAs are expressed in human liver. Proc Natl Acad Sci U S A 1986;83:77-81. [Crossref] [PubMed]
- Goldspink G. Mechanical signals, IGF-I gene splicing, and muscle adaptation. Physiology (Bethesda) 2005;20:232-8. [Crossref] [PubMed]
- Milingos DS, Philippou A, Armakolas A, et al. Insulinlike growth factor-1Ec (MGF) expression in eutopic and ectopic endometrium: characterization of the MGF E-peptide actions in vitro. Mol Med Camb Mass 2011;17:21-8. [Crossref] [PubMed]
- Armakolas A, Philippou A, Panteleakou Z, et al. Preferential expression of IGF-1Ec (MGF) transcript in cancerous tissues of human prostate: evidence for a novel and autonomous growth factor activity of MGF E peptide in human prostate cancer cells. Prostate 2010;70:1233-42. [Crossref] [PubMed]
- Shang J, Fan X, Liu H. The role of mechano-growth factor E peptide in the regulation of osteosarcoma. Oncol Lett 2015;10:697-704. [Crossref] [PubMed]
- Philippou A, Armakolas A, Panteleakou Z, et al. IGF1Ec expression in MG-63 human osteoblast-like osteosarcoma cells. Anticancer Res 2011;31:4259-65. [PubMed]
- Armakolas A, Dimakakos A, Loukogiannaki C, et al. IL-6 is associated to IGF-1Ec upregulation and Ec peptide secretion, from prostate tumors. Mol Med Camb Mass 2018;24:6. [Crossref] [PubMed]
- Wallis M. New insulin-like growth factor (IGF)-precursor sequences from mammalian genomes: the molecular evolution of IGFs and associated peptides in primates. Growth Horm IGF Res 2009;19:12-23. [Crossref] [PubMed]
- Clemmons DR. Insulin-like growth factor binding proteins and their role in controlling IGF actions. Cytokine Growth Factor Rev 1997;8:45-62. [Crossref] [PubMed]
- Pivonello C, De Martino MC, Negri M, et al. The GH-IGF-SST system in hepatocellular carcinoma: biological and molecular pathogenetic mechanisms and therapeutic targets. Infect Agent Cancer 2014;9:27. [Crossref] [PubMed]
- Baxter RC, Bayne ML, Cascieri MA. Structural determinants for binary and ternary complex formation between insulin-like growth factor-I (IGF-I) and IGF binding protein-3. J Biol Chem 1992;267:60-5. [PubMed]
- Ranke MB. Insulin-like growth factor binding-protein-3 (IGFBP-3). Best Pract Res Clin Endocrinol Metab 2015;29:701-11. [Crossref] [PubMed]
- Bikle DD, Tahimic C, Chang W, et al. Role of IGF-I signaling in muscle bone interactions. Bone 2015;80:79-88. [Crossref] [PubMed]
- Baxter RC. Insulin-like growth factor (IGF)-binding proteins: interactions with IGFs and intrinsic bioactivities. Am J Physiol Endocrinol Metab 2000;278:E967-76. [Crossref] [PubMed]
- Rosenfeld RG, Hwa V, Wilson E, et al. The insulin-like growth factor-binding protein superfamily. Growth Horm IGF Res 2000;10 Suppl A:S16-7.
- Hwa V, Oh Y, Rosenfeld RG. The insulin-like growth factor-binding protein (IGFBP) superfamily. Endocr Rev 1999;20:761-87. c. [PubMed]
- Denley A, Cosgrove LJ, Booker GW, et al. Molecular interactions of the IGF system. Cytokine Growth Factor Rev 2005;16:421-39. [Crossref] [PubMed]
- Belfiore A, Malaguarnera R, Vella V, et al. Insulin Receptor Isoforms in Physiology and Disease: An Updated View. Endocr Rev 2017;38:379-431. [Crossref] [PubMed]
- Wang LM, Myers MG, Sun XJ, et al. IRS-1: essential for insulin- and IL-4-stimulated mitogenesis in hematopoietic cells. Science 1993;261:1591-4. [Crossref] [PubMed]
- Shelton JG, Steelman LS, White ER, et al. Synergy between PI3K/Akt and Raf/MEK/ERK pathways in IGF-1R mediated cell cycle progression and prevention of apoptosis in hematopoietic cells. Cell Cycle 2004;3:372-9. [PubMed]
- Shiratsuchi I, Akagi Y, Kawahara A, et al. Expression of IGF-1 and IGF-1R and their relation to clinicopathological factors in colorectal cancer. Anticancer Res 2011;31:2541-5. [PubMed]
- Gydee H, O’Neill JT, Patel A, et al. Differentiated thyroid carcinomas from children and adolescents express IGF-I and the IGF-I receptor (IGF-I-R). Cancers with the most intense IGF-I-R expression may be more aggressive. Pediatr Res 2004;55:709-15. [Crossref] [PubMed]
- Frasca F, Pandini G, Sciacca L, et al. The role of insulin receptors and IGF-I receptors in cancer and other diseases. Arch Physiol Biochem 2008;114:23-37. [Crossref] [PubMed]
- Braulke T. Type-2 IGF receptor: a multi-ligand binding protein. Horm Metab Res 1999;31:242-6. [Crossref] [PubMed]
- von Horn H, Ekström C, Ellis E, et al. GH is a regulator of IGF2 promoter-specific transcription in human liver. J Endocrinol 2002;172:457-65. [Crossref] [PubMed]
- Forbes K, Westwood M. The IGF axis and placental function. a mini review. Horm Res 2008;69:129-37. [PubMed]
- Scott CD, Firth SM. The role of the M6P/IGF-II receptor in cancer: tumor suppression or garbage disposal? Horm Metab Res 2004;36:261-71. [Crossref] [PubMed]
- Harris LK, Westwood M. Biology and significance of signalling pathways activated by IGF-II. Growth Factors 2012;30:1-12. [Crossref] [PubMed]
- Laviola L, Natalicchio A, Giorgino F. The IGF-I signaling pathway. Curr Pharm Des 2007;13:663-9. [Crossref] [PubMed]
- O’Connor JC, McCusker RH, Strle K, et al. Regulation of IGF-I function by proinflammatory cytokines: at the interface of immunology and endocrinology. Cell Immunol 2008;252:91-110. [Crossref] [PubMed]
- Alpdogan O, Muriglan SJ, Kappel BJ, et al. Insulin-like growth factor-I enhances lymphoid and myeloid reconstitution after allogeneic bone marrow transplantation. Transplantation 2003;75:1977-83. [Crossref] [PubMed]
- Baudler S, Baumgartl J, Hampel B, et al. Insulin-like growth factor-1 controls type 2 T cell-independent B cell response. J Immunol 2005;174:5516-25. [Crossref] [PubMed]
- Hunt P, Eardley DD. Suppressive effects of insulin and insulin-like growth factor-1 (IGF1) on immune responses. J Immunol 1986;136:3994-9. [PubMed]
- Frauwirth KA, Thompson CB. Regulation of T lymphocyte metabolism. J Immunol 2004;172:4661-5. [Crossref] [PubMed]
- Tapson VF, Boni-Schnetzler M, Pilch PF, et al. Structural and functional characterization of the human T lymphocyte receptor for insulin-like growth factor I in vitro. J Clin Invest 1988;82:950-7. [Crossref] [PubMed]
- Long E, Huynh HT, Zhao X. Involvement of insulin-like growth factor-1 and its binding proteins in proliferation and differentiation of murine bone marrow-derived macrophage precursors. Endocrine 1998;9:185-92. [Crossref] [PubMed]
- Kooijman R, Coppens A, Hooghe-Peters E. Igf-I inhibits spontaneous apoptosis in human granulocytes. Endocrinology 2002;143:1206-12. [Crossref] [PubMed]
- Cirillo F, Lazzeroni P, Sartori C, et al. Inflammatory Diseases and Growth: Effects on the GH-IGF Axis and on Growth Plate. Int J Mol Sci 2017;18. [PubMed]
- Smolen JS, Aletaha D, McInnes IB. Rheumatoid arthritis. Lancet 2016;388:2023-38. [Crossref] [PubMed]
- Smolen JS, Aletaha D, Koeller M, et al. New therapies for treatment of rheumatoid arthritis. Lancet 2007;370:1861-74. [Crossref] [PubMed]
- McInnes IB, Schett G. The pathogenesis of rheumatoid arthritis. N Engl J Med 2011;365:2205-19. [Crossref] [PubMed]
- Kourilovitch M, Galarza-Maldonado C, Ortiz-Prado E. Diagnosis and classification of rheumatoid arthritis. J Autoimmun 2014;48-49:26-30. [Crossref] [PubMed]
- Paleolog EM. The vasculature in rheumatoid arthritis: cause or consequence? Int J Exp Pathol 2009;90:249-61. [Crossref] [PubMed]
- Neidel J, Blum WF, Schaeffer HJ, et al. Elevated levels of insulin-like growth factor (IGF) binding protein-3 in rheumatoid arthritis synovial fluid inhibit stimulation by IGF-I of articular chondrocyte proteoglycan synthesis. Rheumatol Int 1997;17:29-37. [Crossref] [PubMed]
- Griffin DJ, Ortved KF, Nixon AJ, et al. Mechanical properties and structure-function relationships in articular cartilage repaired using IGF-I gene-enhanced chondrocytes. J Orthop Res 2016;34:149-53. [Crossref] [PubMed]
- Matsumoto T, Tsurumoto T. Inappropriate serum levels of IGF-I and IGFBP-3 in patients with rheumatoid arthritis. Rheumatology (Oxford) 2002;41:352-3. [Crossref] [PubMed]
- Neidel J. Changes in systemic levels of insulin-like growth factors and their binding proteins in patients with rheumatoid arthritis. Clin Exp Rheumatol 2001;19:81-4. [PubMed]
- Matsumoto T, Yamashita S, Rosenfeld RG. Increased levels of IGF-I and IGFBP-3 in synovial fluids of patients with rheumatoid arthritis. Endocr J 1998;45 Suppl:S141-4. [Crossref] [PubMed]
- Matsumoto T, Gargosky SE, Iwasaki K, et al. Identification and characterization of insulin-like growth factors (IGFs), IGF-binding proteins (IGFBPs), and IGFBP proteases in human synovial fluid. J Clin Endocrinol Metab 1996;81:150-5. [PubMed]
- Fernihough JK, Billingham ME, Cwyfan-Hughes S, et al. Local disruption of the insulin-like growth factor system in the arthritic joint. Arthritis Rheum 1996;39:1556-65. [Crossref] [PubMed]
- Boström EA, Svensson M, Andersson S, et al. Resistin and insulin/insulin-like growth factor signaling in rheumatoid arthritis. Arthritis Rheum 2011;63:2894-904. [Crossref] [PubMed]
- Denko CW, Boja B, Moskowitz RW. Growth factors, insulin-like growth factor-1 and growth hormone, in synovial fluid and serum of patients with rheumatic disorders. Osteoarthritis Cartilage 1996;4:245-9. [PubMed]
- Toussirot E, Nguyen NU, Dumoulin G, et al. Relationship between growth hormone-IGF-I-IGFBP-3 axis and serum leptin levels with bone mass and body composition in patients with rheumatoid arthritis. Rheumatology (Oxford) 2005;44:120-5. [Crossref] [PubMed]
- Lee SD, Chen LM, Kuo WW, et al. Serum insulin-like growth factor-axis and matrix metalloproteinases in patients with rheumatic arthritis or rheumatic heart disease. Clin Chim Acta 2006;367:62-8. [Crossref] [PubMed]
- Lemmey A, Maddison P, Breslin A, et al. Association between insulin-like growth factor status and physical activity levels in rheumatoid arthritis. J Rheumatol 2001;28:29-34. [PubMed]
- Alunno A, Bistoni O, Manetti M, et al. Insulin-Like Growth Factor Binding Protein 6 in Rheumatoid Arthritis: A Possible Novel Chemotactic Factor? Front Immunol 2017;8:554. [Crossref] [PubMed]
- Dhaunsi GS, Uppal SS, Haider MZ. Insulin-like growth factor-1 gene polymorphism in rheumatoid arthritis patients. Scand J Rheumatol 2012;41:421-5. [Crossref] [PubMed]
- Erlandsson MC, Töyrä Silfverswärd S, Nadali M, et al. IGF-1R signalling contributes to IL-6 production and T cell dependent inflammation in rheumatoid arthritis. Biochim Biophys Acta Mol Basis Dis 2017;1863:2158-70. [Crossref] [PubMed]
- Laurberg TB, Ellingsen T, Thorsen J, et al. Insulin-like growth factor I receptor density on CD4+T-lymphocytes from active early steroid- and DMARD-naïve rheumatoid arthritis patients is up-regulated and not influenced by 1 year of clinically effective treatment. Rheumatol Int 2012;32:501-4. [Crossref] [PubMed]
- Abdi E, Najafipour H, Joukar S, et al. Expression of IGF-1, IL-27 and IL-35 Receptors in Adjuvant Induced Rheumatoid Arthritis Model. Iran J Immunol IJI 2018;15:14-27. [PubMed]
- Fulci V, Scappucci G, Sebastiani GD, et al. miR-223 is overexpressed in T-lymphocytes of patients affected by rheumatoid arthritis. Hum Immunol 2010;71:206-11. [Crossref] [PubMed]
- Lu MC, Yu CL, Chen HC, et al. Increased miR-223 expression in T cells from patients with rheumatoid arthritis leads to decreased insulin-like growth factor-1-mediated interleukin-10 production. Clin Exp Immunol 2014;177:641-51. [Crossref] [PubMed]
- Aziz F. The emerging role of miR-223 as novel potential diagnostic and therapeutic target for inflammatory disorders. Cell Immunol 2016;303:1-6. [Crossref] [PubMed]
- Elkan A-C, Håkansson N, Frostegård J, et al. Rheumatoid cachexia is associated with dyslipidemia and low levels of atheroprotective natural antibodies against phosphorylcholine but not with dietary fat in patients with rheumatoid arthritis: a cross-sectional study. Arthritis Res Ther 2009;11:R37. [Crossref] [PubMed]
- Engvall I-L, Elkan A-C, Tengstrand B, et al. Cachexia in rheumatoid arthritis is associated with inflammatory activity, physical disability, and low bioavailable insulin-like growth factor. Scand J Rheumatol 2008;37:321-8. [Crossref] [PubMed]
- Baker JF, Von Feldt JM, Mostoufi-Moab S, et al. Insulin-like Growth Factor 1 and Adiponectin and Associations with Muscle Deficits, Disease Characteristics, and Treatments in Rheumatoid Arthritis. J Rheumatol 2015;42:2038-45. [Crossref] [PubMed]
- Lemmey AB, Marcora SM, Chester K, et al. Effects of high-intensity resistance training in patients with rheumatoid arthritis: a randomized controlled trial. Arthritis Rheum 2009;61:1726-34. [Crossref] [PubMed]
- Karatay S, Yildirim K, Melikoglu MA, et al. Effects of dynamic exercise on circulating IGF-1 and IGFBP-3 levels in patients with rheumatoid arthritis or ankylosing spondylitis. Clin Rheumatol 2007;26:1635-9. [Crossref] [PubMed]
- Melikoglu MA, Karatay S, Senel K, et al. Association between dynamic exercise therapy and IGF-1 and IGFBP-3 concentrations in the patients with rheumatoid arthritis. Rheumatol Int 2006;26:309-13. [Crossref] [PubMed]
- Li J, Xu B, Wu C, et al. TXNDC5 contributes to rheumatoid arthritis by down-regulating IGFBP1 expression. Clin Exp Immunol 2018;192:82-94. [Crossref] [PubMed]
- Shoop-Worrall SJW, Kearsley-Fleet L, Thomson W, et al. How common is remission in juvenile idiopathic arthritis: A systematic review. Semin Arthritis Rheum 2017;47:331-7. [Crossref] [PubMed]
- Ravelli A, Martini A. Juvenile idiopathic arthritis. Lancet 2007;369:767-78. [Crossref] [PubMed]
- Guszczyn T, Rzeczycka J, Popko J. IGF-I and IGF-binding proteins in articular exudates of children with post-traumatic knee damage and juvenile idiopathic arthritis. Pathobiology 2009;76:260-6. [Crossref] [PubMed]
- Davies UM, Jones J, Reeve J, et al. Juvenile rheumatoid arthritis. Effects of disease activity and recombinant human growth hormone on insulin-like growth factor 1, insulin-like growth factor binding proteins 1 and 3, and osteocalcin. Arthritis Rheum 1997;40:332-40. [Crossref] [PubMed]
- De Benedetti F, Meazza C, Oliveri M, et al. Effect of IL-6 on IGF binding protein-3: a study in IL-6 transgenic mice and in patients with systemic juvenile idiopathic arthritis. Endocrinology 2001;142:4818-26. [Crossref] [PubMed]
- Bjørnhart B, Juul A, Nielsen S, et al. Cartilage oligomeric matrix protein in patients with juvenile idiopathic arthritis: relation to growth and disease activity. J Rheumatol 2009;36:1749-54. [Crossref] [PubMed]
- Woo PM. Growth retardation and osteoporosis in juvenile chronic arthritis. Clin Exp Rheumatol 1994;12 Suppl 10:S87-90. [PubMed]
- Bilginer Y, Topaloglu R, Alikasifoglu A, et al. Low cortisol levels in active juvenile idiopathic arthritis. Clin Rheumatol 2010;29:309-14. [Crossref] [PubMed]
- Wong SC, MacRae VE, Gracie JA, et al. Inflammatory cytokines in juvenile idiopathic arthritis: effects on physical growth and the insulin-like-growth factor axis. Growth Horm IGF Res 2008;18:369-78. [Crossref] [PubMed]
- Tsatsoulis A, Siamopoulou A, Petsoukis C, et al. Study of growth hormone secretion and action in growth-retarded children with juvenile chronic arthritis (JCA). Growth Horm IGF Res 1999;9:143-9. [Crossref] [PubMed]
- Lewander P, Dahle C, Larsson B, et al. Circulating cartilage oligomeric matrix protein in juvenile idiopathic arthritis. Scand J Rheumatol 2017;46:194-7. [Crossref] [PubMed]
- Bechtold S, Ripperger P, Mühlbayer D, et al. GH therapy in juvenile chronic arthritis: results of a two-year controlled study on growth and bone. J Clin Endocrinol Metab 2001;86:5737-44. [Crossref] [PubMed]
- Bozzola E, Pagani S, Meazza C, et al. Changes in growth hormone receptor gene expression during therapy in children with juvenile idiopathic arthritis. Horm Res Paediatr 2012;77:52-8. [Crossref] [PubMed]
- Clemmons DR, Jones JI, Busby WH, et al. Role of insulin-like growth factor binding proteins in modifying IGF actions. Ann N Y Acad Sci 1993;692:10-21. [Crossref] [PubMed]
- Lelbach A, Muzes G, Feher J. The insulin-like growth factor system: IGFs, IGF-binding proteins and IGFBP-proteases. Acta Physiol Hung 2005;92:97-107. [Crossref] [PubMed]
- Schmeling H, Seliger E, Horneff G. Growth reconstitution in juvenile idiopathic arthritis treated with etanercept. Clin Exp Rheumatol 2003;21:779-84. [PubMed]
- De Benedetti F, Brunner H, Ruperto N, et al. Catch-up growth during tocilizumab therapy for systemic juvenile idiopathic arthritis: results from a phase III trial. Arthritis Rheumatol 2015;67:840-8. [Crossref] [PubMed]
- Ghodke-Puranik Y, Niewold TB. Immunogenetics of systemic lupus erythematosus: A comprehensive review. J Autoimmun 2015;64:125-36. [Crossref] [PubMed]
- Dörner T, Lipsky PE. Beyond pan-B-cell-directed therapy - new avenues and insights into the pathogenesis of SLE. Nat Rev Rheumatol 2016;12:645-57. [Crossref] [PubMed]
- Denko CW, Malemud CJ. Age-related changes in serum growth hormone, insulin-like growth factor-1 and somatostatin in system lupus erythematosus. BMC Musculoskelet Disord 2004;5:37. [Crossref] [PubMed]
- Waldron J, Raymond W, Ostli-Eilertsen G, et al. Insulin-like growth factor-1 (IGF1) in systemic lupus erythematosus: relation to disease activity, organ damage and immunological findings. Lupus 2018;27:963-70. [Crossref] [PubMed]
- Mok CC, Ding HH, Kharboutli M, et al. Axl, Ferritin, IGFBP2 and TNFR2 as biomarkers in systemic lupus erythematosus. Arthritis Care Res 2016;68:1303-9. [Crossref]
- Wu T, Xie C, Han J, et al. Insulin-Like Growth Factor Binding Protein-4 as a Marker of Chronic Lupus Nephritis. PloS One 2016;11:e0151491. [Crossref] [PubMed]
- Mohammed JA, Mok AYP, Parbtani A, et al. Increased expression of insulin-like growth factors in progressive glomerulonephritis of the MRL/lpr mouse. Lupus 2003;12:584-90. [Crossref] [PubMed]
- Ding H, Kharboutli M, Saxena R, et al. Insulin-like growth factor binding protein-2 as a novel biomarker for disease activity and renal pathology changes in lupus nephritis. Clin Exp Immunol 2016;184:11-8. [Crossref] [PubMed]
- Stanilova SA, Ivanova MG, Karakolev IA, et al. Association of +3179G/A insulin-like growth factor-1 receptor polymorphism and insulin-like growth factor-1 serum level with systemic lupus erythematosus. Lupus 2013;22:1388-93. [Crossref] [PubMed]
- Fawzi MMT, Tawfik SO, Eissa AM, et al. Expression of insulin-like growth factor-I in lesional and non-lesional skin of patients with morphoea. Br J Dermatol 2008;159:86-90. [Crossref] [PubMed]
- Hamaguchi Y, Fujimoto M, Matsushita T, et al. Elevated serum insulin-like growth factor (IGF-1) and IGF binding protein-3 levels in patients with systemic sclerosis: possible role in development of fibrosis. J Rheumatol 2008;35:2363-71. [Crossref] [PubMed]
- Hsu E, Feghali-Bostwick CA. Insulin-like growth factor-II is increased in systemic sclerosis-associated pulmonary fibrosis and contributes to the fibrotic process via Jun N-terminal kinase- and phosphatidylinositol-3 kinase-dependent pathways. Am J Pathol 2008;172:1580-90. [Crossref] [PubMed]
- Rothe MJ, Altman RD, Falanga V. Plasma somatomedin-C levels in systemic sclerosis. Br J Dermatol 1988;119:639-42. [Crossref] [PubMed]
- Winsz-Szczotka K, Kuźnik-Trocha K, Komosińska-Vassev K, et al. Relationship between adiponectin, leptin, IGF-1 and total lipid peroxides plasma concentrations in patients with systemic sclerosis: possible role in disease development. Int J Rheum Dis 2016;19:706-14. [Crossref] [PubMed]
- Emamian ES, Leon JM, Lessard CJ, et al. Peripheral blood gene expression profiling in Sjögren’s syndrome. Genes Immun 2009;10:285-96. [Crossref] [PubMed]
- Markopoulos AK, Poulopoulos AK, Kayavis I, et al. Immunohistochemical detection of insulin-like growth factor-I in the labial salivary glands of patients with Sjögren’s syndrome. Oral Dis 2000;6:31-4. [Crossref] [PubMed]
- Gu YS, Kong J, Cheema GS, et al. The immunobiology of systemic sclerosis. Semin Arthritis Rheum 2008;38:132-60. [Crossref] [PubMed]
- Stern EP, Denton CP. The Pathogenesis of Systemic Sclerosis. Rheum Dis Clin North Am 2015;41:367-82. [Crossref] [PubMed]
- Lasky JA, Brody AR. Interstitial fibrosis and growth factors. Environ Health Perspect 2000;108 Suppl 4:751-62. [PubMed]
- Steen VD, Medsger TA. Changes in causes of death in systemic sclerosis, 1972-2002. Ann Rheum Dis 2007;66:940-4. [Crossref] [PubMed]
- Harrison NK, Cambrey AD, Myers AR, et al. Insulin-like growth factor-I is partially responsible for fibroblast proliferation induced by bronchoalveolar lavage fluid from patients with systemic sclerosis. Clin Sci 1994;86:141-8. [Crossref] [PubMed]
- Moutsopoulos HM. Sjögren’s syndrome: autoimmune epithelitis. Clin Immunol Immunopathol 1994;72:162-5. [Crossref] [PubMed]
- Kerr M, Lee A, Wang PL, et al. Detection of insulin and insulin-like growth factors I and II in saliva and potential synthesis in the salivary glands of mice: Effects of type 1 diabetes mellitus. Biochem Pharmacol 1995;49:1521-31. [Crossref] [PubMed]
- Meyer S, Chibly AM, Burd R, et al. Insulin-Like Growth Factor-1-Mediated DNA Repair in Irradiated Salivary Glands Is Sirtuin-1 Dependent. J Dent Res 2017;96:225-32. [Crossref] [PubMed]
- Kobayashi S, Kamino Y, Hiratsuka K, et al. Age-related changes in IGF-1 expression in submandibular glands of senescence-accelerated mice. J Oral Sci 2004;46:119-25. [Crossref] [PubMed]
- Mitsui R, Fujita-Yoshigaki J, Narita T, et al. Maintenance of paracellular barrier function by insulin-like growth factor-I in submandibular gland cells. Arch Oral Biol 2010;55:963-9. [Crossref] [PubMed]
- Mustafa W, Mustafa A, Elbakri N, et al. Reduced levels of insulin-like growth factor-1 receptor (IGF-1R) suppress cellular signaling in experimental autoimmune sialadenitis (EAS). J Recept Signal Transduct Res 2001;21:47-54. [Crossref] [PubMed]
Cite this article as: Skarlis C, Nezos A, Mavragani CP, Koutsilieris M. The role of insulin growth factors in autoimmune diseases. Ann Res Hosp 2019;3:10.